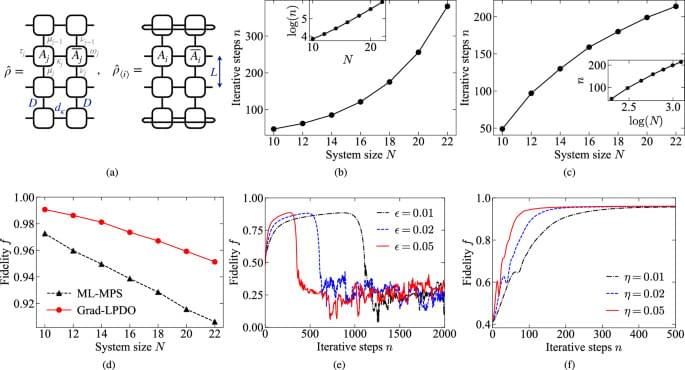
Quantum state tomography plays a fundamental role in characterizing and evaluating the quality of quantum states produced by quantum devices. It serves as a crucial element in the advancement of quantum hardware and software, regardless of the underlying physical implementation and potential applications1,2,3. However, reconstructing the full quantum state becomes prohibitively expensive for large-scale quantum systems that exhibit potential quantum advantages4,5, as the number of measurements required increases exponentially with system size.
Recent protocols try to solve this challenge through two main steps: efficient parameterization of quantum states and utilization of carefully designed measurement schemes and classical data postprocessing algorithms. For one-dimensional (1D) systems with area law entanglement, the matrix product state (MPS)6,7,8,9,10,11,12 provides a compressed representation. It requires only a polynomial number of parameters that can be determined from local or global measurement results. Two iterative algorithms using local measurements, singular value thresholding (SVT)13 and maximum likelihood (ML)14, have been demonstrated in trapped-ion quantum simulators with up to 14 qubits15. However, SVT is limited to pure states and thus impractical for noisy intermediate-scale quantum (NISQ) systems. Meanwhile, although ML can handle mixed states represented as matrix product operators (MPOs)16,17, it suffers from inefficient classical data postprocessing.