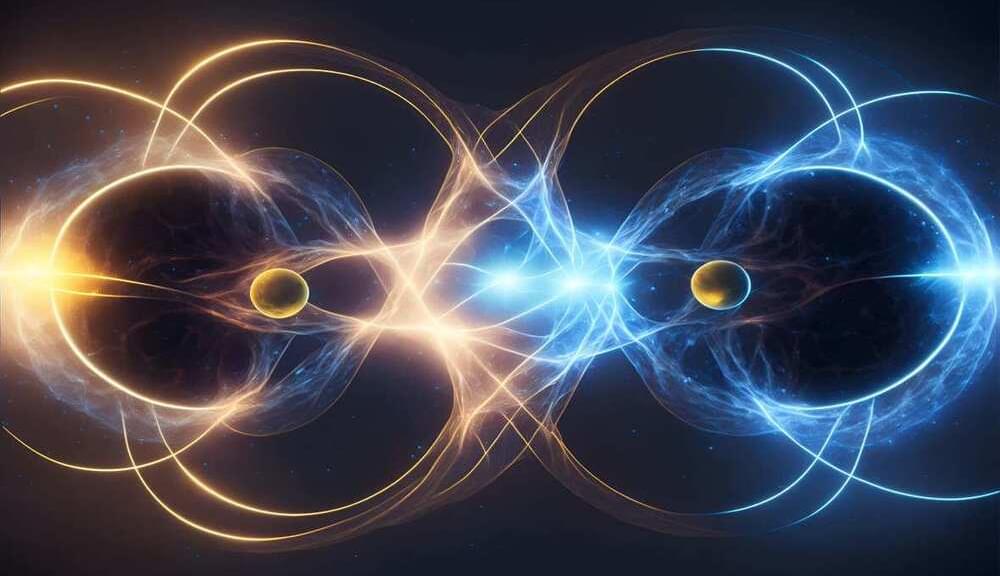
Quantum mechanics, a realm of the incredibly small, is often characterized by its paradoxical nature. One such paradox is the concept of superposition, where a quantum particle can exist in multiple states simultaneously. These delicate states, however, are notoriously fragile, often collapsing into a single, definite state within mere fractions of a second. Yet, a recent breakthrough has pushed the boundaries of quantum stability, achieving a record-breaking 23-minute lifespan for a specific type of superposition known as a cat state.
The term “cat state” is a whimsical reference to Schrödinger’s famous thought experiment, where a cat is placed in a box with a device that could randomly kill it. Until the box is opened, the cat is both alive and dead, a superposition of two states. In quantum mechanics, cat states manifest when a quantum object, such as an atom or a photon, exists in multiple states simultaneously, defying classical intuition.
While researchers have previously created cat states in laboratories, these states have been fleeting, quickly succumbing to the disruptive influence of their environment. However, a team led by Zheng-Tian Lu at the University of Science and Technology of China has managed to extend the lifespan of a cat state dramatically. They achieved this feat by manipulating a cloud of 10,000 ytterbium atoms, cooled to near absolute zero and trapped by laser light. By carefully controlling the atoms’ quantum states, the researchers were able to induce a superposition where each atom existed in two distinct spin states simultaneously.