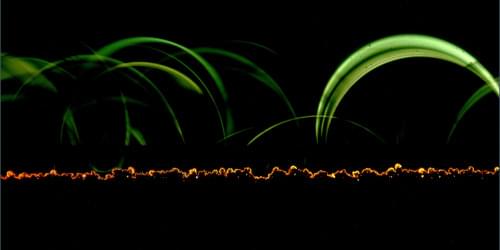
The discovery of a mini aurora above a light-emitting polymer material reveals an electron-ejection process that might be useful in field-emission displays and material fabrication.
Auroras occur in the night sky when charged solar-wind particles, such as protons and electrons, are deflected by Earth’s magnetic field and interact with molecules in the atmosphere. Researchers have now found an aurora-like emission coming from a light-emitting polymer [1]. The surprising display consisted of flashes of green light above the polymer surface. The researchers explained the emission as the result of electrons being ejected from the polymer and interacting with a vapor of organic molecules. The discovery suggests that these polymers might be useful as electron emitters for applications such as spectroscopy, medical technology, and lithography.
Jun Gao from Queen’s University in Canada is amazed by auroras, and he’s even gone out on cold nights to look for them. But he was not prepared for the aurora that showed up in his lab two years ago. He and his student at the time, Dongze Wang, were testing failure modes for polymer light-emitting electrochemical cells, or PLECs, used in light sources and display devices. These cells are organic semiconductors that are electrochemically doped on one side to have excess electrons (making an n-type semiconductor) and on the other side to have electron deficiencies, or holes (making a p-type semiconductor). Electrons crossing the p – n boundary can fill holes and produce red light.