A new study has identified how a common brain parasite, Toxoplasma gondii, significantly disrupts brain function by affecting the number of extracellular vesicles (EVs) released by neurons.
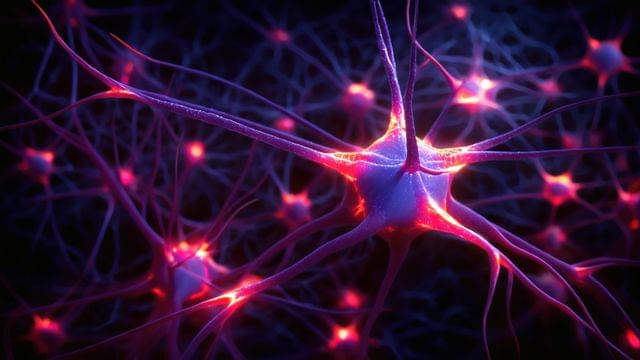
Super Humanity — Imagine if your brain could interface directly with AI.
Super Humanity explores the revolutionary intersection of neuroscience and technology, revealing a future where artificial intelligence integrates effortlessly with human thought.
Super Humanity (2019)
Director: Ruth Chao.
Writers: Ruth Chao, Paula Cons, Alphonse de la Puente.
Genre: Documentary, Sci-Fi.
Country: Portugal, Spain.
Language: English.
Release Date: December 27, 2019 (Spain)
Synopsis:
The convergence of human brains and AI will create a new breed of humanity—often described as ‘super-humanity.’
By enabling brain-machine interfaces, human cognitive powers will be amplified, marking the dawn of enhanced humans. Connected minds will unlock advanced synthetic telepathy, offering not only the ability to perceive others’ thoughts but also to influence them. Yet, what are the advantages and dangers posed by these groundbreaking advancements?
Neurotechnology stands at the threshold of a societal transformation, reshaping our concepts of identity and reality itself. The establishment of neuro-rights will be crucial, requiring laws that protect the privacy of our conscious and even subconscious minds.
Mind Forward delves deeply into the potential of this new frontier.
Macquarie University researchers have discovered a naturally occurring protein found in human cells plays a powerful role in repairing damaged DNA — the molecule that carries the genetic instructions for building and maintaining living things.
The discovery, published in the journal Ageing Cell, could hold the key to developing therapies for devastating age-related diseases such as motor neuron disease (MND), Alzheimer’s disease, and Parkinson’s disease.
Hope: Dr Sina Shadfar, pictured, and colleagues discovered a protein which they have shown for the first time acts like a ‘glue’, helping to repair broken DNA, which is widely accepted as one of the main contributors to ageing and the progression of age-related diseases.
The research, conducted by neurobiologist Dr Sina Shadfar and colleagues in the Motor Neuron Disease Research Centre, reveals a protein called protein disulphide isomerase (PDI) helps repair serious deoxyribonucleic acid (DNA) damage. This breakthrough opens new possibilities for therapies aimed at boosting the body’s ability to fix its own DNA — a process that becomes less efficient as we age.
A new study published in Proceedings of the National Academy of Sciences reveals that chronic exposure to artificial light at night (LAN) can trigger depression-like behaviors by activating a specific neural pathway in the brain.
The study, conducted on tree shrews—diurnal mammals genetically close to primates that are active during the day like humans—offers critical insights into how nighttime light may disrupt mood regulation, potentially affecting human mental health in increasingly illuminated urban environments.
The research team, led by Prof. Xue Tian from the University of Science and Technology of China (USTC), Prof. Yao Yonggang of the Kunming Institute of Zoology of the Chinese Academy of Sciences (CAS), and Prof. Zhao Huan of Hefei University, exposed tree shrews to blue light (comparable to bright indoor lighting) for two hours each night for three weeks.
You wouldn’t microwave fish around your worst enemy—the smell lingers both in kitchen and memory. It is one few of us like, let alone have positive associations with. But what makes our brains decide a smell is stinky?
A new study from UF Health researchers reveals the mechanisms behind how your brain decides you dislike—even loathe—a smell. The findings are published in the journal Molecular Psychiatry.
Or as first author and graduate research fellow Sarah Sniffen puts it: How do odors come to acquire some sort of emotional charge?