3D-printed middle ear transplant, Prof. Mashudu Tshifularo, hearing loss treatment, medical innovation, middle ear surgery, 3D printing in medicine
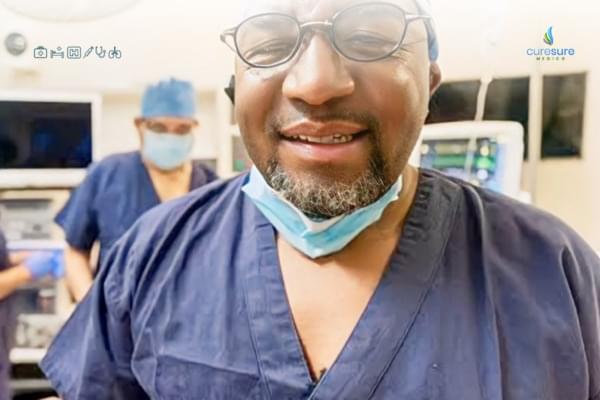
What if reversing cellular aging could be achieved by overexpressing just a single gene? In this video, I break down Shift Bioscience’s latest preprint, whic…
New research from Harvard T.H. Chan School of Public Health and Ben Gurion University in Israel reveals that nearly one-third of people who stuck to a healthy diet didn’t lose any weight, but still saw impressive health improvements.
Even without shedding pounds, participants experienced key benefits to their cardiometabolic health. These included higher levels of HDL cholesterol (often called the “good” cholesterol), reduced levels of leptin (a hormone that drives hunger), and less visceral fat, which is the deep belly fat that can surround vital organs.
“We have been conditioned to equate weight loss with health, and weight loss-resistant individuals are often labeled as failures,” said lead author Anat Yaskolka Meir, postdoctoral research fellow in the Department of Epidemiology at Harvard Chan School. “Our findings reframe how we define clinical success. People who do not lose weight can improve their metabolism and reduce their long-term risk for disease. That’s a message of hope, not failure.”
Scientists from the A*STAR Genome Institute of Singapore (A*STAR GIS) have developed a new artificial intelligence (AI)-based method called “Fragle” that makes tracking cancer easier and faster using blood tests.
Requiring only a small blood sample, this method analyzes the size of DNA fragments in the blood to reveal distinct patterns that differentiate cancer DNA from healthy DNA, helping doctors track cancer treatment response more accurately and frequently. The research was published in Nature Biomedical Engineering in March 2025.
Existing methods for measuring cancer DNA in the blood, also known as circulating tumor DNA (ctDNA), often require complex and expensive DNA sequencing to screen for common cancer mutations. However, because cancer mutations vary between patients, test results can be inconsistent, making it difficult for doctors to track cancer treatment response with blood tests effectively.
An international study has, for the first time, revealed a strong and direct link between the rise of antibiotic-resistant bacteria and universal disinfection procedures applied to patients in intensive care units. Published in The Lancet Microbe, the study calls for a reassessment of health care guidelines on the widespread use of disinfectants.
“Our research highlights the unintended consequences of universal decolonization in a global context where antibiotic resistance is an increasing threat,” says Marco Oggioni, professor at the Department of Pharmacy and Biotechnology at the University of Bologna and one of the study’s authors. “Coordinated efforts to prevent antibiotic-resistant infections are crucial, but they must not prevent us from critically re-evaluating the tools we use to achieve these goals.”
Universal decolonization is a preventive procedure applied to patients upon admission to intensive care. The entire body is disinfected with chlorhexidine—a commonly used antiseptic also employed to sanitize medical devices and hospital surfaces—and an additional nasal treatment is administered with another disinfectant, mupirocin.
Physical reservoir computing refers to the concept of using nonlinear physical systems as computational resources to achieve complex information processing. This approach exploits the intrinsic properties of physical systems such as their nonlinearity and memory to perform computational tasks. Soft biological tissues possess characteristics such as stress-strain nonlinearity and viscoelasticity that satisfy the requirements of physical reservoir computing. This study evaluates the potential of human soft biological tissues as physical reservoirs for information processing. Particularly, it determines the feasibility of using the inherent dynamics of human soft tissues as a physical reservoir to emulate nonlinear dynamic systems. In this concept, the deformation field within the muscle, which is obtained from ultrasound images, represented the state of the reservoir. The findings indicate that the dynamics of human soft tissue have a positive impact on the computational task of emulating nonlinear dynamic systems. Specifically, our system outperformed the simple LR model for the task. Simple LR models based on raw inputs, which do not account for the dynamics of soft tissue, fail to emulate the target dynamical system (relative error on the order of <inline-formula xmlns:mml=“http://www.w3.org/1998/Math/MathML” xmlns:xlink=“http://www.w3.org/1999/xlink”> <tex-math notation=“LaTeX”>$10^{-2}$ </tex-math></inline-formula>). By contrast, the emulation results obtained using our system closely approximated the target dynamics (relative error on the order of <inline-formula xmlns:mml=“http://www.w3.org/1998/Math/MathML” xmlns:xlink=“http://www.w3.org/1999/xlink”> <tex-math notation=“LaTeX”>$10^{-3}$ </tex-math></inline-formula>). These results suggest that the soft tissue dynamics contribute to the successful emulation of the nonlinear equation. This study suggests that human soft tissues can be used as a potential computational resource. Soft tissues are found throughout the human body. Therefore, if computational processing is delegated to biological tissues, it could lead to a distributed computation system for human-assisted devices.
Dr. Mohammed Enayat has access to all sorts of experimental antiaging treatments at his clinic, but a core part of his longevity routine is pretty cheap and accessible: supplements.
Enayat told Business Insider that his most recent “biological age” tests, taken 18 months ago, said he was 24, or 17 years younger than his chronological age of 41. There’s no consensus on how to define or measure biological age, but Enayat used GlycanAge and TruAge PACE, which measure inflammation and epigenetics, respectively.
The primary care doctor, who’s also the founder of London’s Hum2n longevity clinic, has been closely tracking his health for the past seven years, using wearable tech, including an Oura ring and a Whoop strap, plus regular blood, urine, and microbiome tests.
As neuro-ophthalmology educators, we have sought ways to improve the teaching of pupil-related disorder, focusing on incorporating their dynamic aspects and active learning. Our solution is an app for smartphone and tablet devices. The app, Pupil Wizard, provides a digital textbook featuring a dynamic presentation of the key pupillary abnormalities. It allows the users to interact with a digital patient and explore how each condition responds to direct and indirect light stimuli, near focus, and changes in ambient light (Fig. 1). Moreover, the users can test their knowledge in quiz mode, where random pupillary abnormalities must be correctly identified and multiple-choice questions about them answered.
Open Access This article is licensed under a Creative Commons Attribution 4.0 International License, which permits use, sharing, adaptation, distribution and reproduction in any medium or format, as long as you give appropriate credit to the original author(s) and the source, provide a link to the Creative Commons licence, and indicate if changes were made. The images or other third party material in this article are included in the article’s Creative Commons licence, unless indicated otherwise in a credit line to the material. If material is not included in the article’s Creative Commons licence and your intended use is not permitted by statutory regulation or exceeds the permitted use, you will need to obtain permission directly from the copyright holder. To view a copy of this licence, visit http://creativecommons.org/licenses/by/4.0/.