Discover the fifth state of matter, beyond solid, liquid, gas, and plasma. This is a quantum leap in physics with groundbreaking scientific potential.
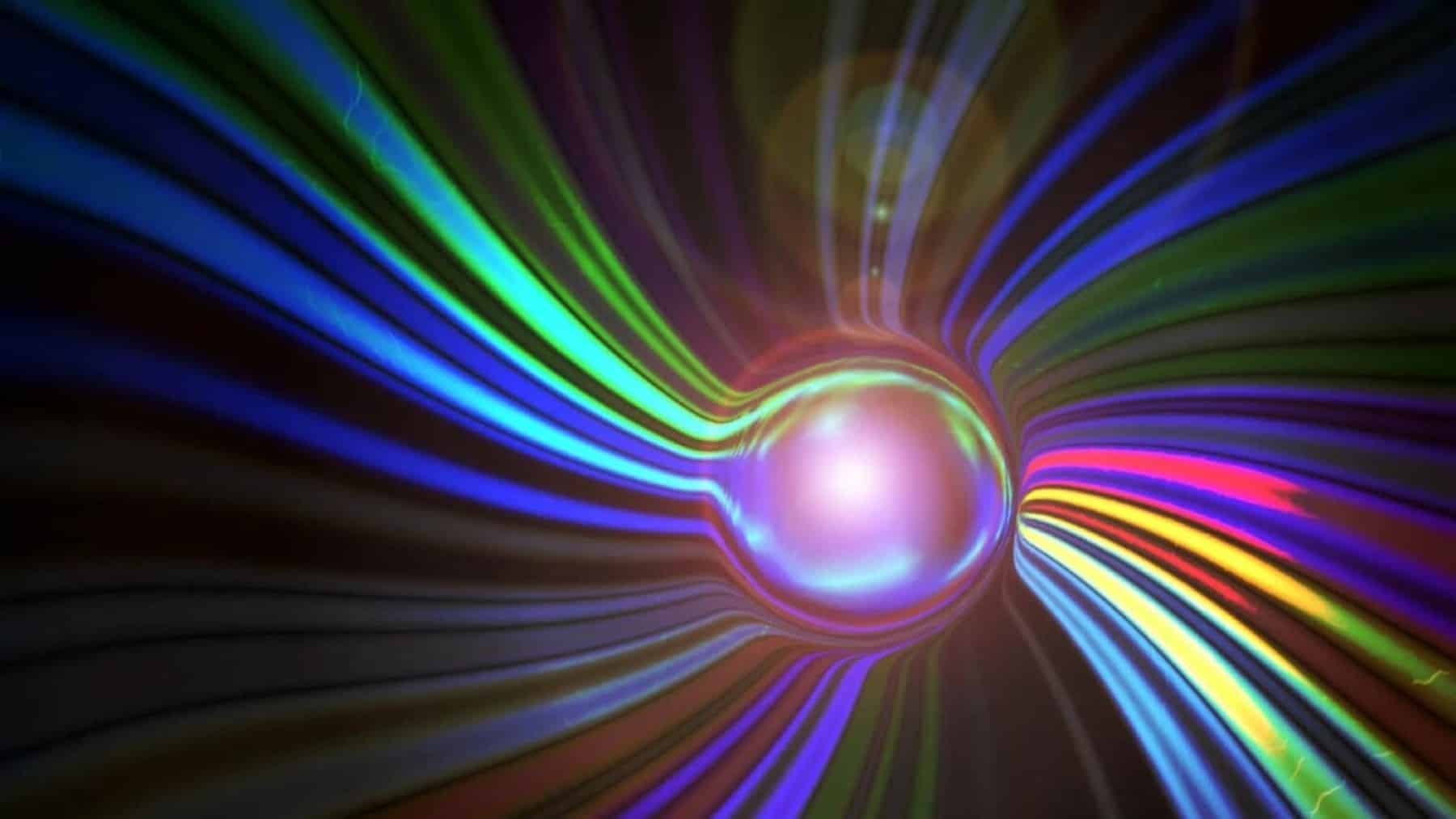
Sounds like magic, works like physics.
Tête de Moine, a semi-hard Swiss cheese that often finds its way onto charcuterie boards and salads, not only brings a rich, nutty and creamy flavor, but also adds a dramatic flare to the presentation. Instead of slicing, this cheese is shaved into delicate rosettes using a tool called a Girolle whose rotating blade gently scrapes thin layers of cheese into ruffled curls. These pretty cheese flowers are known to enhance the flavor and texture due to their high surface-to-volume ratio.
The unusual way Tête de Moine forms wrinkles when shaved, piqued the interest of a team of physicists who, in a study published in Physical Review Letters, set out to investigate the physical mechanisms behind these intricate shapes.
Similar morphogenetic patterns can be observed in the frilly edges of leaves, fungi, corals, or even torn plastic sheets, but the mechanisms that explain the similar shapes in these materials fail to account for the distinctive physical properties of cheese.
The researcher added that with better data on the horizon, including the first public data on galaxy clustering from DESI released last week, the team will re-apply their methods, compare their results with their current findings, and detect any statistically significant differences.
“I think there are more questions than answers at this point,” Chen said. “This research certainly enforces the idea that different cosmological datasets are beginning to be in tension when interpreted within the standard Λ CDM model of cosmology.”
Almost every galaxy hosts a supermassive black hole at its center. When galaxies merge, the two black holes spiral in closer to each other and eventually merge through gravitational-wave emission. Within a few billion years, this process will be featured close to home as our own Milky-Way will collide with its nearest massive neighbor, the Andromeda galaxy.
If the two black holes have different masses, the emission of gravitational waves is asymmetric, causing the merger product to recoil. The intense burst of gravitational waves in a preferred direction during the final plunge of the two black holes towards each other, kicks the remnant black hole in the opposite direction through the rocket effect. The end result is that gravitational waves propel the black hole remnant to speeds of up to a few percent of the speed of light. The recoiling black hole behaves like the payload of a rocket powered by gravitational waves.
In 2007, I published a single-authored paper in the prestigious journal Physical Review Letters, suggesting that a gravitational-wave recoil could displace a black hole from the galactic center and endow it with fast motion relative to the background stars. If the kick is modest, dynamical friction on the background gas or stars would eventually return the black hole back to the center.
Kirigami is a traditional Japanese art form that entails cutting and folding paper to produce complex three-dimensional (3D) structures or objects. Over the past decades, this creative practice has also been applied in the context of physics, engineering, and materials science research to create new materials, devices and even robotic systems.
Researchers at Sichuan University and McGill University recently devised a new approach for the inverse engineering of kirigami, which does not rely on advanced computational tools and numerical algorithms. This new method, outlined in a paper published in Physical Review Letters, could simplify the design of intricate kirigami for a wide range of real-world applications.
“This work is a natural extension of our previous work on kirigami,” Damiano Pasini, senior corresponding author of the paper, told Phys.org.
Monte Carlo methods, or Monte Carlo experiments, are a broad class of computational algorithms that rely on repeated random sampling to obtain numerical results. The underlying concept is to use randomness to solve problems that might be deterministic in principle. The name comes from the Monte Carlo Casino in Monaco, where the primary developer of the method, mathematician Stanisław Ulam, was inspired by his uncle’s gambling habits.
Monte Carlo methods are mainly used in three distinct problem classes: optimization, numerical integration, and generating draws from a probability distribution. They can also be used to model phenomena with significant uncertainty in inputs, such as calculating the risk of a nuclear power plant failure. Monte Carlo methods are often implemented using computer simulations, and they can provide approximate solutions to problems that are otherwise intractable or too complex to analyze mathematically.
Monte Carlo methods are widely used in various fields of science, engineering, and mathematics, such as physics, chemistry, biology, statistics, artificial intelligence, finance, and cryptography. They have also been applied to social sciences, such as sociology, psychology, and political science. Monte Carlo methods have been recognized as one of the most important and influential ideas of the 20th century, and they have enabled many scientific and technological breakthroughs.
Quasars represent some of the most luminous and energetic phenomena in the universe. These distant powerhouses are driven by supermassive black holes—colossal gravitational engines with masses millions to billions of times that of our sun—which actively devour surrounding matter at incredible rates.
As gas, dust, and stellar material spiral inward through an accretion disk superheated to millions of degrees, this matter releases tremendous energy across the electromagnetic spectrum before crossing the event horizon. The resulting emissions can outshine entire galaxies despite originating from a region no larger than our solar system.
The discovery of billion-solar-mass black holes in distant quasars challenges conventional growth models in astrophysics. Scientists have observed these supermassive black holes (SMBHs) at redshifts beyond z≳6, when the universe was less than a billion years old—theoretically insufficient time for them to reach such enormous masses through standard Eddington-limited accretion from stellar-mass seeds.