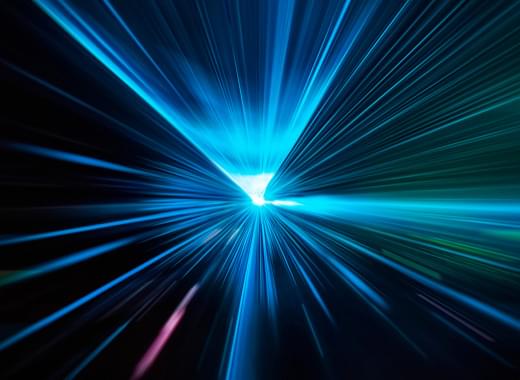
In 2019, researchers from the Massachusetts Institute of Technology made headlines when they created the “blackest black” material made from carbon nanotubes —ten times blacker than any material that had been manufactured at that time—a material so black that it had the ability to absorb 99.995% of incident light. Such research in light absorption is not a trivial pursuit or mere aesthetics, there are many technologies that can benefit from maximizing light absorption—for instance, in photovoltaics because of the need to absorb and convert as much light as possible into electricity, or on the interior surface of a light sensor because of the need to minimize unwanted stray light. The physics of light absorption can get quite complex when you get into the details, as what we non-technically consider as “black” is usually not a perfect absorber. Indeed, there are many ways to create something that can absorb some light, but the endeavor gets increasingly more difficult the closer one attempts to achieve 100% absorption.
That takes some serious physics.
Now, physicists in Austria and Israel report in the journal Science that they have engineered a light trap that utilizes the quantum properties of electromagnetic waves— in which waveforms undergo constructive or destructive interference when combined in just the right manner—to generate an anti-laser that has near-perfect light absorption [1]. Because the light trap functions essentially as a time-reversed laser, where instead of multiple passes of single-wavelength light for maximum stimulated emission of photons the multiple passes are engineered for maximum absorption, the device is a veritable anti-laser.