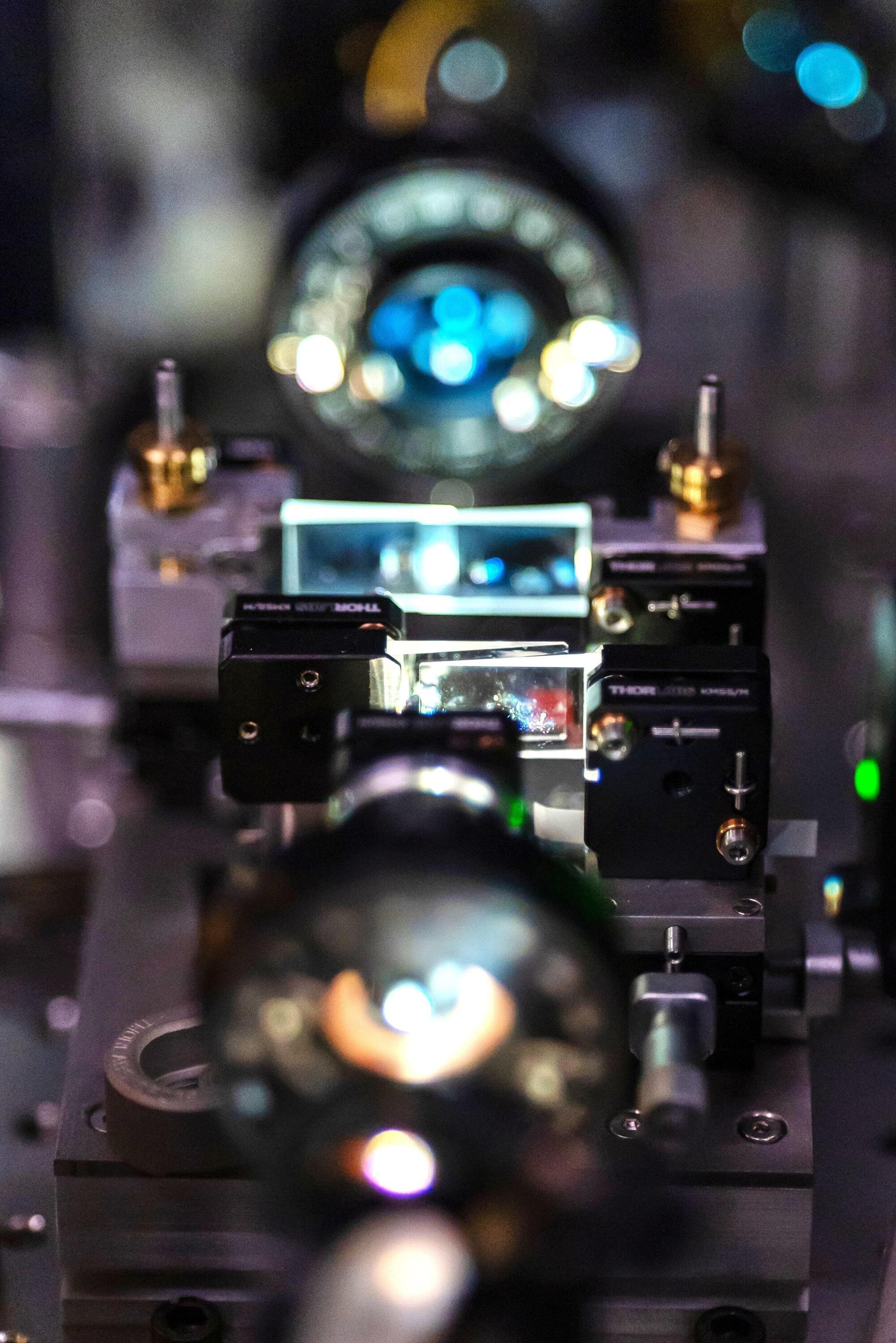
The ultrafast dynamics and interactions of electrons in molecules and solids have long remained hidden from direct observation. For some time now, it has been possible to study these quantum-physical processes—for example, during chemical reactions, the conversion of sunlight into electricity in solar cells and elementary processes in quantum computers—in real time with a temporal resolution of a few femtoseconds (quadrillionths of a second) using two-dimensional electronic spectroscopy (2DES).
However, this technique is highly complex. Consequently, it has only been employed by a handful of research groups worldwide to date. Now a German-Italian team led by Prof. Dr. Christoph Lienau from the University of Oldenburg has discovered a way to significantly simplify the experimental implementation of this procedure. “We hope that 2DES will go from being a methodology for experts to a tool that can be widely used,” explains Lienau.
Two doctoral students from Lienau’s Ultrafast Nano-Optics research group, Daniel Timmer and Daniel Lünemann, played a key role in the discovery of the new method. The team has now published a paper in Optica describing the procedure.