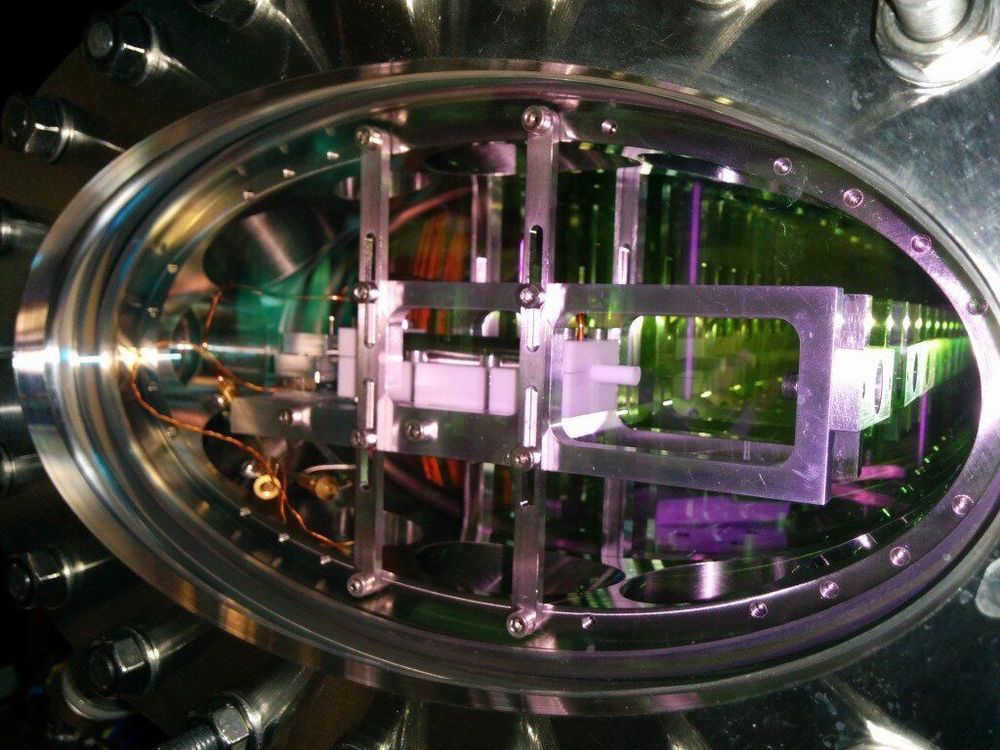
A team of physicists led by Professor Patrick Windpassinger at Johannes Gutenberg University Mainz (JGU) has successfully transported light stored in a quantum memory over a distance of 1.2 millimeters. They have demonstrated that the controlled transport process and its dynamics has only little impact on the properties of the stored light. The researchers used ultra-cold rubidium-87 atoms as a storage medium for the light as to achieve a high level of storage efficiency and a long lifetime.
“We stored the light by putting it in a suitcase so to speak, only that in our case the suitcase was made of a cloud of cold atoms. We moved this suitcase over a short distance and then took the light out again. This is very interesting not only for physics in general, but also for quantum communication, because light is not very easy to ‘capture’, and if you want to transport it elsewhere in a controlled manner, it usually ends up being lost,” said Professor Patrick Windpassinger, explaining the complicated process.
The controlled manipulation and storage of quantum information as well as the ability to retrieve it are essential prerequisites for achieving advances in quantum communication and for performing corresponding computer operations in the quantum world. Optical quantum memories, which allow for the storage and on-demand retrieval of quantum information carried by light, are essential for scalable quantum communication networks. For instance, they can represent important building blocks of quantum repeaters or tools in linear quantum computing. In recent years, ensembles of atoms have proven to be media well suited for storing and retrieving optical quantum information. Using a technique known as electromagnetically induced transparency (EIT), incident light pulses can be trapped and coherently mapped to create a collective excitation of the storage atoms. Since the process is largely reversible, the light can then be retrieved again with high efficiency.