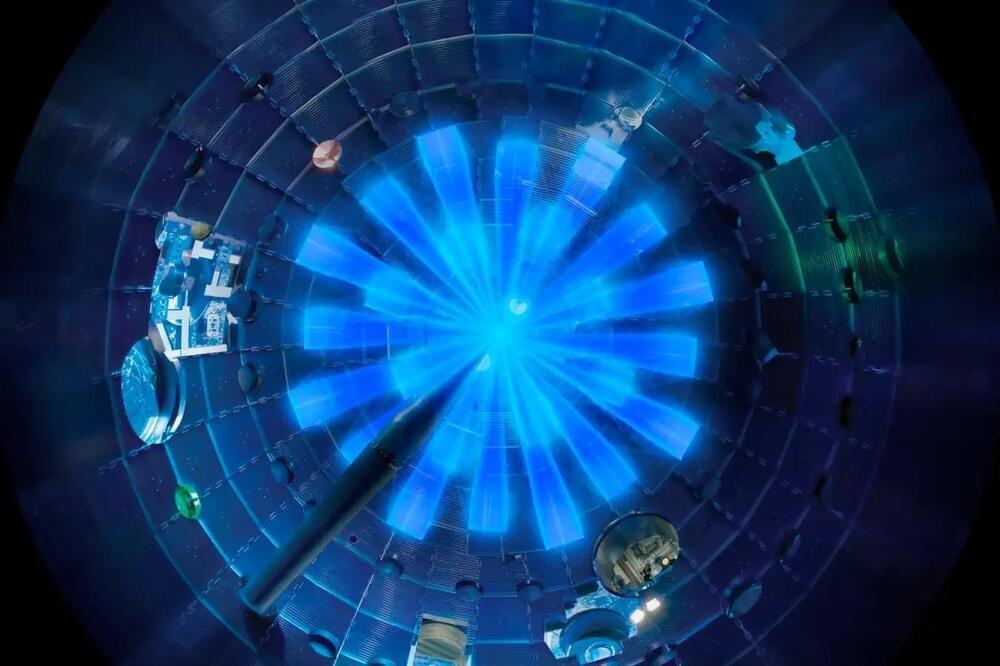
Scientists achieved a record-breaking 10 quadrillion-watt energy burst using 192 giant lasers.
Researchers at the Lawrence Livermore National Laboratory in California have achieved a groundbreaking result in nuclear fusion by generating an energy burst of more than 10 quadrillion watts. This was accomplished by using 192 giant lasers to target a tiny hydrogen pellet, releasing 1.3 megajoules of energy in a fraction of a second. The experiment, carried out at the National Ignition Facility (NIF), marks a significant step forward in fusion research and brings scientists closer to achieving “ignition,” where a fusion reaction generates more energy than it consumes.
In this latest experiment, conducted at the NIF, researchers focused intense beams of light from the world’s largest lasers onto a pea-sized pellet of hydrogen. The lasers delivered an immense amount of energy to the pellet, causing it to emit 1.3 megajoules of energy in just 100 trillionths of a second. This amount of energy is equivalent to about 10% of the sunlight that hits Earth at any moment and is significantly higher than the previous record of 170 kilojoules.
Although the hydrogen pellet absorbed more energy from the lasers than it released, the experiment produced approximately 70% of the energy absorbed, a dramatic improvement over past efforts. Scientists hope to eventually reach the break-even point, where the fusion reaction releases 100% or more of the energy it absorbs.