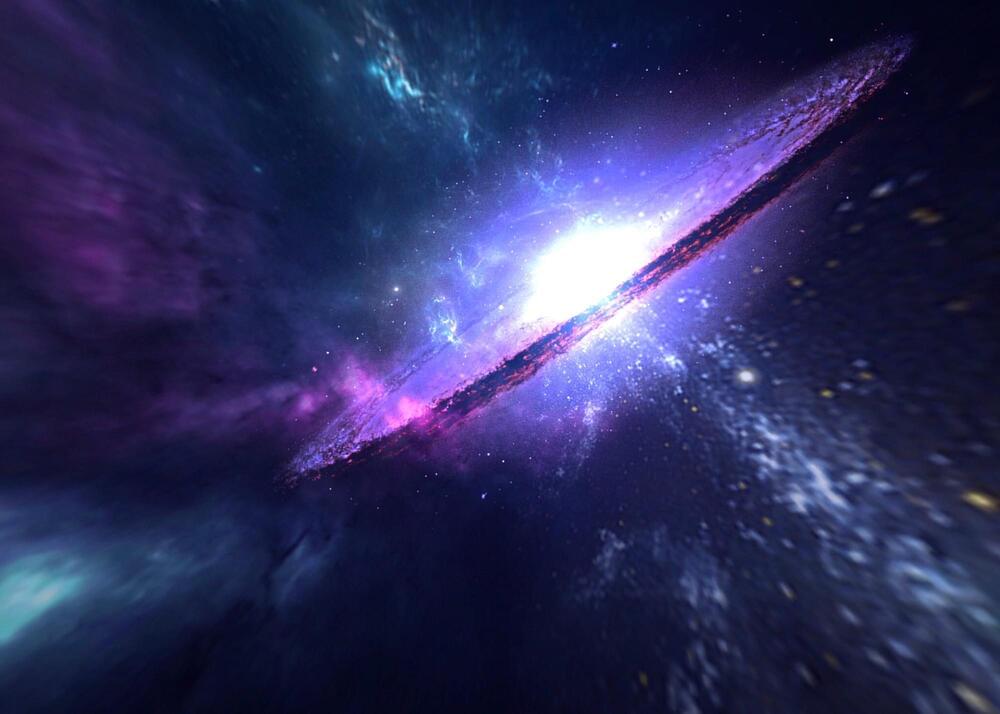
A new hypothesis suggests that the very fabric of space-time may act as a dynamic reservoir for quantum information, which, if it holds, would address the long-standing Black Hole Information Paradox and potentially reshape our understanding of quantum gravity, according to a research team including scientists from pioneering quantum computing firm, Terra Quantum and Leiden University.
Published in Entropy, the Quantum Memory Matrix (QMM) hypothesis offers a mathematical framework to reconcile quantum mechanics and general relativity while preserving the fundamental principle of information conservation.
The study proposes that space-time, quantized at the Planck scale — a realm where the physics of quantum mechanics and general relativity converge — stores information from quantum interactions in “quantum imprints.” These imprints encode details of quantum states and their evolution, potentially enabling information retrieval during black hole evaporation through mechanisms like Hawking radiation. This directly addresses the Black Hole Information Paradox, which highlights the conflict between quantum mechanics — suggesting information cannot be destroyed — and classical black hole descriptions, where information appears to vanish once the black hole evaporates.