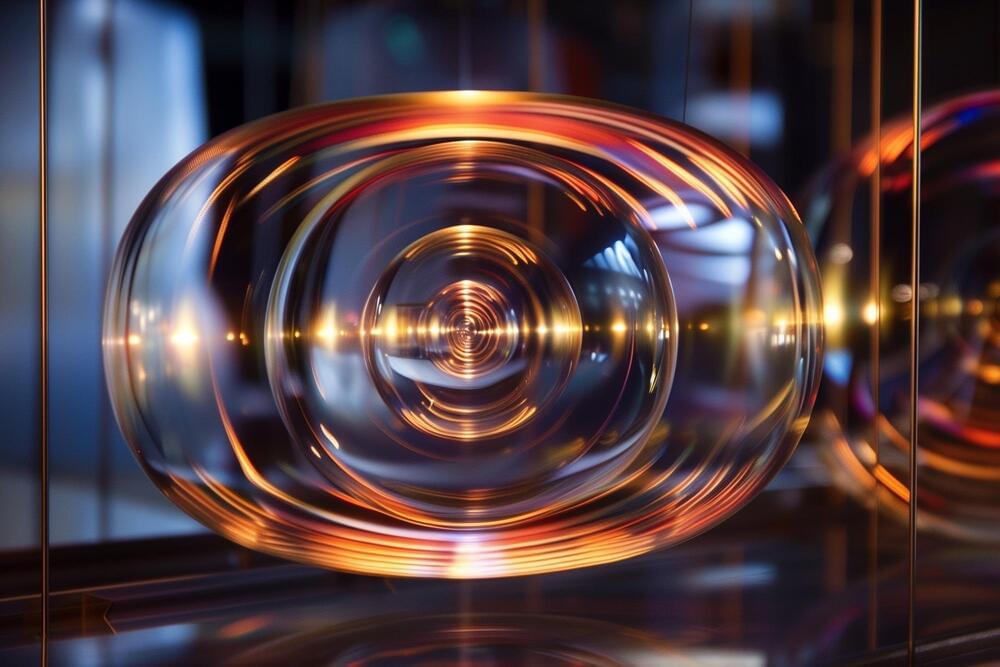
Quantum squeezing is a method that sharpens precision by redistributing uncertainty within a system, already advancing technologies like atomic clocks. This concept promises even wider impacts as researchers work on applying it to more complex measurements.
Quantum squeezing is a technique in quantum physics that reduces uncertainty in one aspect of a system while increasing it in another. Imagine a balloon filled with air: when it’s untouched, the balloon is perfectly round. If you squeeze one side, it flattens in that spot but stretches in the opposite direction.
Similarly, in a squeezed quantum state, reducing uncertainty (or noise) in one variable, like position, causes increased uncertainty in a related variable, such as momentum. The total uncertainty remains the same, but redistributing it in this way allows for far more precise measurement of one of the variables.