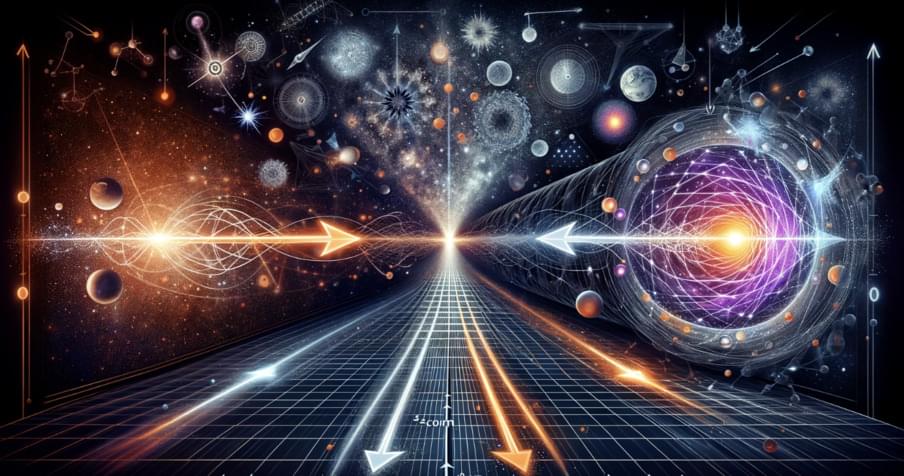
Ask anyone working in quantum computing and they may tell you they have been dealing with the frustratingly contrarian and intricately delicate state of entanglement since the beginning of time. However, a new study suggests this might be impossible. In fact, entanglement may have been absent in the earliest moments of the universe, researchers are reporting — a hypothesis that would — if validated — challenge our understanding of quantum mechanics and the nature of time itself.
The research, detailed in a paper by Jim Al-Khalili, of the University of Surrey and Eddy Keming Chen, University of California, San Diego and published on the pre-print server ArXiv, explores the so-called entanglement past hypothesis. In the study, the researchers explore why time only flows in one direction, a fundamental concept in both quantum physics and thermodynamics.
According to the researchers the concept of quantum entanglement, where two particles become so deeply linked that their properties seem to remain interconnected regardless of the distance between them, is central to modern quantum mechanics. It’s also a key ingredient for the potential of quantum computers to tackle massively complex calculations. It’s also why quantum computing is so vexing, because entanglement can be disrupted by external influences, leading to a process known as decoherence.