A process based on perovskite semiconductors is described to downscale micro-LEDs and nano-LEDs to below the conventional size limits, demonstrating average external quantum efficiencies maintained at around 20% across a wide range of pixel lengths.
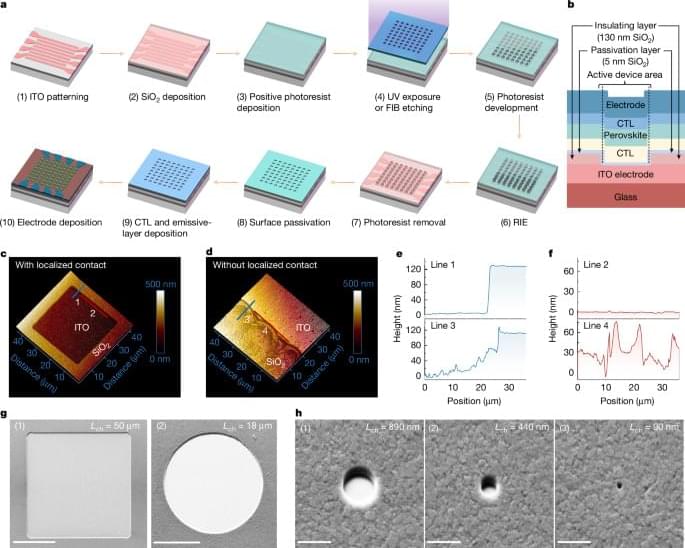
A study led by the University of Portsmouth has achieved unprecedented precision in detecting tiny shifts in light displacements at the nanoscale. This is relevant in the characterization of birefringent materials and in high-precision measurements of rotations.
The quantum sensing breakthrough is published in the journal Physical Review A, and has the potential to revolutionize many aspects of daily life, industry, and science.
Imagine two photons, massless particles of light, that are intertwined in a unique way, meaning their propagation is connected even when they are separated. When these photons pass through a device that splits the particles of light into two paths—known as a beam-splitter—they interfere with each other in special patterns. By analyzing these patterns, researchers have developed a highly precise method to detect even the tiniest initial spatial shifts between them.
Scientists from the RIKEN Center for Emergent Matter Science (CEMS) and collaborators have discovered a new way to control superconductivity—an essential phenomenon for developing more energy-efficient technologies and quantum computing—by simply twisting atomically thin layers within a layered device.
By adjusting the twist angle, they were able to finely tune the “superconducting gap,” which plays a key role in the behavior of these materials. The research is published in Nature Physics.
The superconducting gap is the energy threshold required to break apart Cooper pairs—bound electron pairs that enable superconductivity at low temperatures. Having a larger gap allows superconductivity to persist at higher, more accessible temperatures, and tuning the gap is also important for optimizing Cooper pair behavior at the nanoscale, contributing to the high functionality of quantum devices.
Memory engrams are formed through experience-dependent plasticity of neural circuits, but their detailed architectures remain unresolved. Using three-dimensional electron microscopy, we performed nanoscale reconstructions of the hippocampal CA3-CA1 pathway after chemogenetic labeling of cellular ensembles recruited during associative learning. Neurons with a remote history of activity coinciding with memory acquisition showed no strong preference for wiring with each other. Instead, their connectomes expanded through multisynaptic boutons independently of the coactivation state of postsynaptic partners. The rewiring of ensembles representing an initial engram was accompanied by input-specific, spatially restricted upscaling of individual synapses, as well as remodeling of mitochondria, smooth endoplasmic reticulum, and interactions with astrocytes.
A new study resulting from a collaboration between King Abdullah University of Science and Technology (KAUST) and King Abdulaziz City for Science and Technology (KACST) shows how nanomaterials can significantly reduce the carbon emissions of LED (light-emitting diode) streetlights. The research team estimates that by adopting this technology, the United States alone can reduce carbon dioxide emissions by more than one million metric tons.
The findings are published in the journal Light: Science & Applications.
The nanomaterial, called nanoPE, enhances the emission of thermal radiation from the surface of the LED to reduce the LED temperature. LEDs generate heat, which raises their temperature and risks damaging the LED electronics and shortening the LED’s lifespan. In fact, approximately 75% of the input energy in LEDs is eventually lost as heat.
Scientists have demonstrated how to manipulate light by using time-varying media in the form of specialized nanomaterials.
Peanut butter and jelly. Simon and Garfunkel. Semiconductors and bacteria. Some combinations are more durable than others. In recent years, an interdisciplinary team of Cornell researchers has been pairing microbes with the semiconductor nanocrystals known as quantum dots, with the goal of creating nano-biohybrid systems that can harvest sunlight to perform complex chemical transformations for materials and energy applications.
Now, the team has for the first time identified exactly what happens when a microbe receives an electron from a quantum dot: The charge can either follow a direct pathway or be transferred indirectly via the microbe’s shuttle molecules.
The findings are published in Proceedings of the National Academy of Sciences. The lead author is Mokshin Suri.
When light interacts with metallic nanostructures, it instantaneously generates plasmonic hot carriers, which serve as key intermediates for converting optical energy into high-value energy sources such as electricity and chemical energy. Among these, hot holes play a crucial role in enhancing photoelectrochemical reactions. However, they thermally dissipate within picoseconds (trillionths of a second), making practical applications challenging.
Now, a Korean research team has successfully developed a method for sustaining hot holes longer and amplifying their flow, accelerating the commercialization of next-generation, high-efficiency, light-to-energy conversion technologies.
The research team, led by Distinguished Professor Jeong Young Park from the Department of Chemistry at KAIST, in collaboration with Professor Moonsang Lee from the Department of Materials Science and Engineering at Inha University, has successfully amplified the flow of hot holes and mapped local current distribution in real time, thereby elucidating the mechanism of photocurrent enhancement. The work is published in Science Advances.
A team of scientists has created a chiral assembly by blending inorganic polyoxometalates and organic cyclodextrin molecules.
Polyoxometalates (POMs) are a class of nanomaterials with many useful applications. However, using polyoxometalates as building blocks to construct chiral POM-based frameworks has been a long-standing challenge for researchers. The team produced a 3D framework in this research, constructed by coordination assembly. The resulting framework features an interlaced organic-inorganic hybrid layer.
The team has published their work in the journal Polyoxometalates.
Physicists have measured a nuclear reaction that can occur in neutron star collisions, providing direct experimental data for a process that had previously only been theorized. The study, led by the University of Surrey, provides new insight into how the universe’s heaviest elements are forged—and could even drive advancements in nuclear reactor physics.
Working in collaboration with the University of York, the University of Seville, and TRIUMF, Canada’s national particle accelerator center, the breakthrough marks the first-ever measurement of a weak r-process reaction cross-section using a radioactive ion beam, in this case studying the 94 Sr(α, n)97 Zr reaction. This is where a radioactive form of strontium (strontium-94) absorbs an alpha particle (a helium nucleus), then emits a neutron and transforms into zirconium-97.
The study has been published in Physical Review Letters.