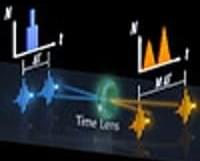
Time-resolved photon counting plays an indispensable role in precision metrology in both classical and quantum regimes. Therein, time-correlated single-photon counting (TCSPC) [1] has been the key enabling technology for applications such as fluorescence lifetime microscopy [2], time-gated Raman spectroscopy [3], photon counting time-of-flight (ToF) 3D imaging [4], light-in-flight imaging [5], and computational diffuse optical tomography [6]. For all these applications, one of the most important figures of merit is the single-photon timing resolution (SPTR, also referred to as photon counting timing jitter). The TCSPC SPTR is limited by the available single-photon detectors. For example, photomultiplier tubes typically provide an SPTR larger than 100 ps [7]. Meanwhile, superconducting nanowire single-photon detectors have superior SPTR in the sub-10-ps range [8, 9]. However, cryogenic cooling significantly increases the system complexity. Single-photon avalanche diodes (SPADs) operate at moderate temperature, which makes them a popular choice for various applications mentioned above. Nevertheless, their SPTR is still limited to tens-of-picoseconds level [10]. On the other hand, orders-of-magnitude enhancement on SPTR is required for many challenging applications such as the study of ultrafast fluorescent decay dynamics [11,12].
In this Letter, we demonstrate a time-magnified TCSPC (TM-TCSPC) that achieves an ultrashort SPTR of 550 fs using an off-the-shelf single-photon detector. The key component is a quantum temporal magnifier using a low-noise high-efficiency fiber parametric time lens [13,14] based on four-wave mixing Bragg scattering (FWM-BS) [15 – 17]. A temporal magnification of 130 with a 97% photon conversion efficiency has been achieved while maintaining the quantum coherence of the signal under test (SUT). Detection sensitivity of -{95}\;rm{dBm}$ (0.03 photons per pulse), limited by the spontaneous Raman scattering noise, is possible and allows efficient processing and characterization of quantum-level SUT. The TM-TCSPC can resolve ultrashort pulses with a 130-fs pulse width difference at a 22-fs accuracy. When applied to photon counting ToF 3D imaging, the TM-TCSPC greatly suppresses the range walk error (RWE) that limits all photon counting ToF 3D imaging systems by 99.2% (130 times) and thus provides high depth measurement accuracy and precision of 26 µm and 3 µm, respectively. The TM-TCSPC is a promising solution for photon counting at the femtosecond regime that will benefit various research fields such as fluorescence lifetime microscopy, time-gated Raman spectroscopy, light-in-flight imaging, and computational diffuse optical tomography.