The senior product manager leading hardware and software product development at the Center for Quantum Computing wants to make fault-tolerant quantum computing a reality.
Category: computing – Page 487
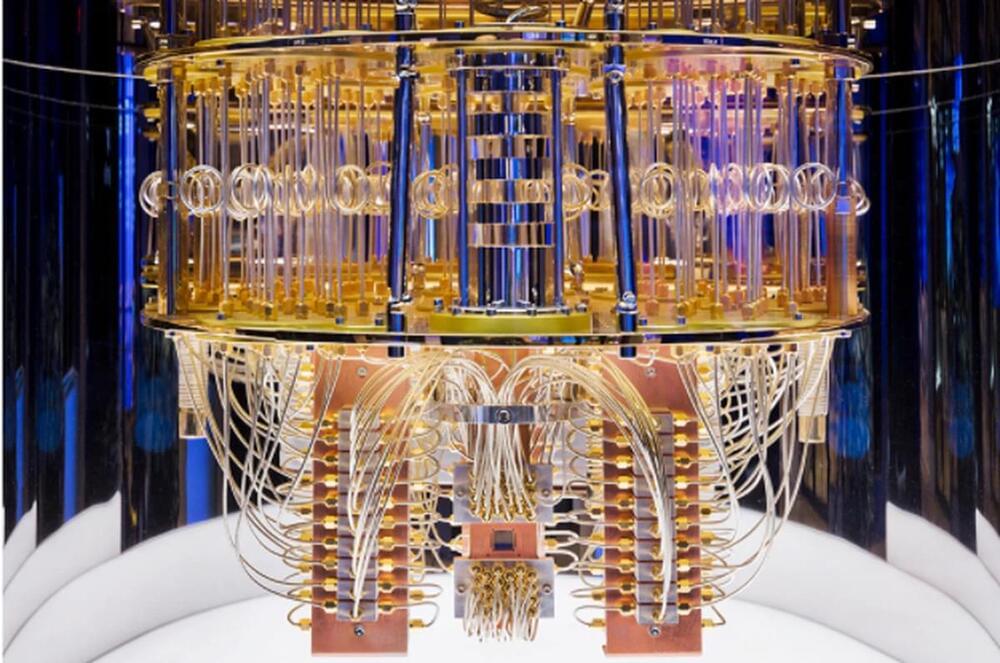

Stretchy light-emitting plastic could be used in wearable screens
An elastic light-emitting polymer that glows like a filament in a light bulb could lead to affordable, practical and robust flexible screens.
Flexible screens could form part of wearable computers that stick to our skin and do away with the need to carry a separate smartphone or laptop. But the various existing flexible displays all have flaws: they either require high voltages to run, are too fragile, too expensive, not bendy enough or lack brightness.
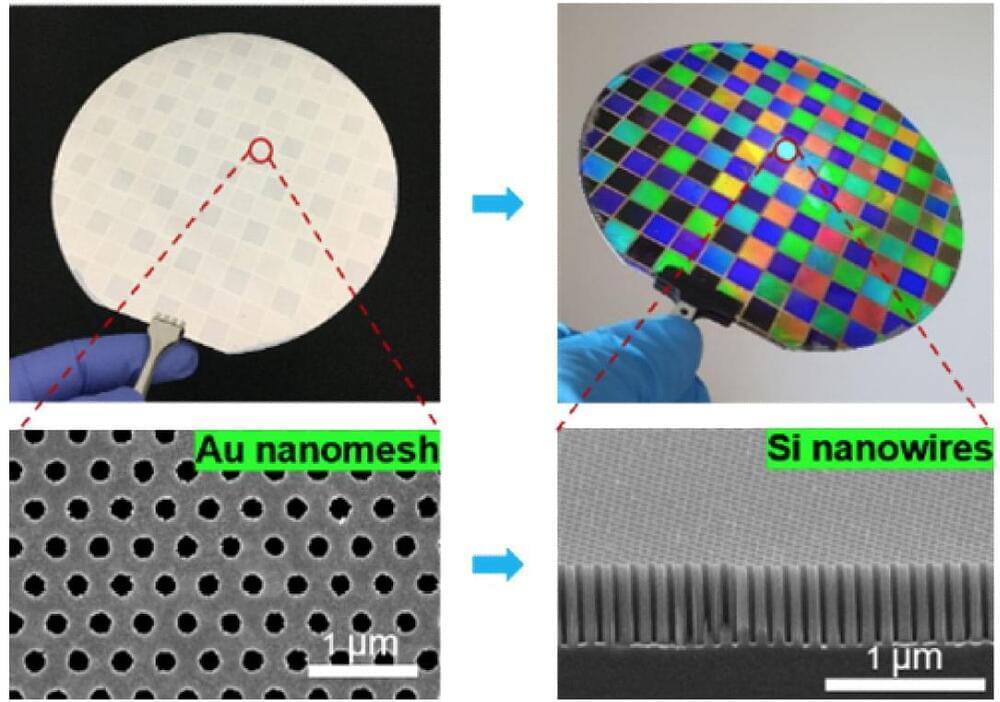
New technique opens door to cheaper semiconductors, higher chip yield
Scientists from the NTU Singapore and the Korea Institute of Machinery & Materials (KIMM) have developed a technique to create a highly uniform and scalable semiconductor wafer, paving the way to higher chip yield and more cost-efficient semiconductors.
Left: Image of a six-inch silicon wafer with printed metal layers and its top-view scanning electron microscope image. Right: Image of the six-inch silicon wafer with nanowires and its cross-sectional scanning electron microscope image. (Image: NTU Singpore)
Semiconductor chips commonly found in smart phones and computers are difficult and complex to make, requiring highly advanced machines and special environments to manufacture.
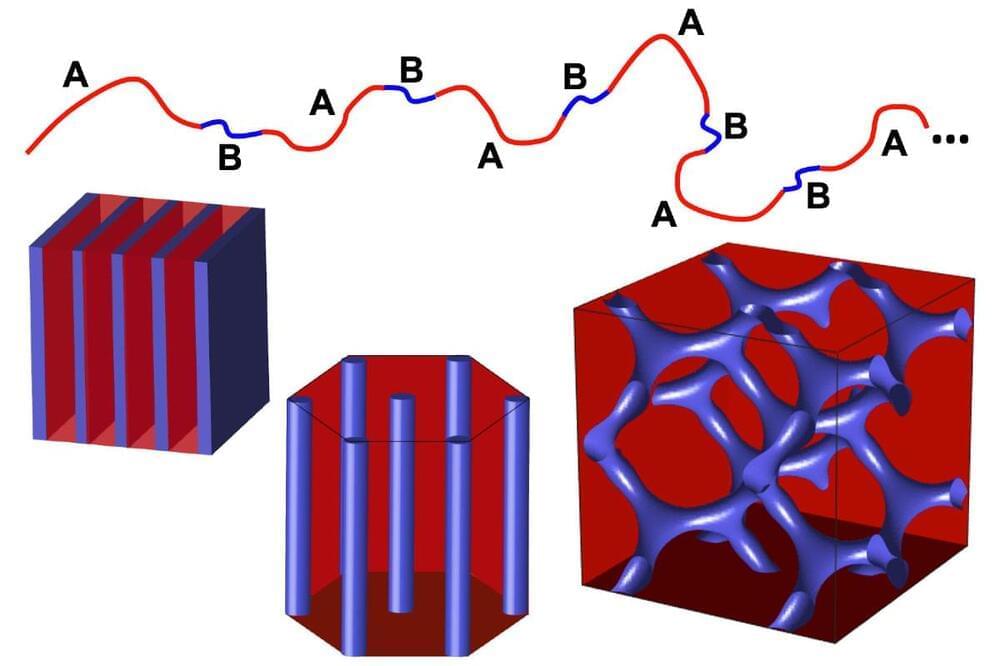
A new class of materials for nanopatterning
The microscopic components that make up computer chips must be made at staggering scales. With billions of transistors in a single processor, each made of multiple materials carefully arranged in patterns as thin as a strand of DNA, their manufacturing tools must also operate at a molecular level.
Typically, these tools involve using stencils to selectively pattern or remove materials with high fidelity, layer after layer, to form nanoscale electronic devices. But as chips must fit more and more components to keep up with the digital world’s growing computational demands, these nanopatterning stencils must also become smaller and more precise.
Now, a team of Penn Engineers has demonstrated how a new class of polymers could do just that. In a new study, the researchers demonstrated how “multiblock” copolymers can produce exceptionally ordered patterns in thin films, achieving spacings smaller than three nanometers.

How Can Quantum Computing Change the World?
Every industry will be affected by quantum computing. They will alter the way business is done and the security systems in place which protect data, how we battle illnesses and create new materials, as well as how we tackle health and climate challenges.
As the race to build the first commercially functional quantum computer heats up, here we discuss a handful of the ways quantum computing will alter our world.
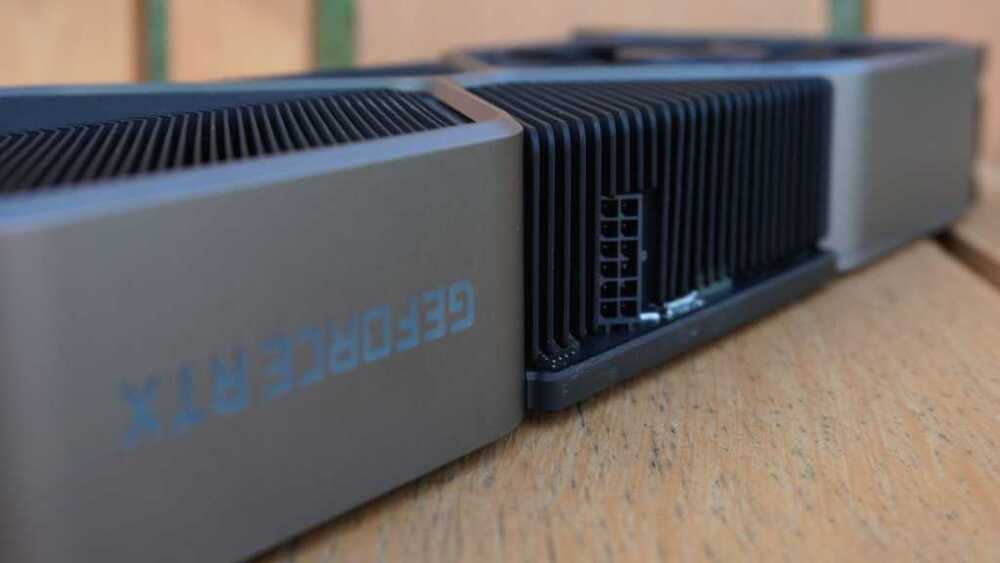
The future of PSUs is here: Intel’s ATX 3.0 powers monster 600W graphics cards
PC power supplies haven’t seen a whole lot of change in the last decade or two. We’ve gotten modular cables for easier routing, smaller standards for itty-bitty builds, and that’s about it. But today Intel has finalized the ATX 3.0 standard, coming soon to a full-sized PC case near you. The biggest addition announced today is a new standardized connection for graphics cards and other PCIe devices, delivering up to 600 watts on a single connector.
Currently graphics cards are in a bit of a power pinch. The maximum throughput for an 8-pin ATX rail is 150 watts, so the biggest and most power-hungry GPUs need to double or even triple up, adding extra space requirements and more complex cable routing inside the case. The new 12-pin 12VHPWR connection should be able to deliver more energy than even the most powerful graphics cards need for the next generation or two. Each pin housing is also physically smaller, with a 3.0mm pitch versus 4.2mm on current power supply rails.
Technically it’s 16 total pins (12+4), with four additional data pins squeezed in beneath the primary power pins. This is to manage DC output voltage regulation and a series of new tools designed to regulate high power output efficiently and safely, all handled intelligently by the power supply. According to Intel, the new 12VHPWR connection will be the standard for “most, if not all” PCIe cards using the 5.0 spec.

Direct generation of complex structured light
Extension of laser beam structures promises new laser applications. Exploration of how beam structures change during nonlinear frequency conversion processes has drawn increasing interest in recent years. Nonlinear conversion is an excellent route for structured beam generation and represents a growing, hybrid field for researchers in nonlinear optics and laser technology, as well as the emerging area of light-field regulation technology.
For structured beam generation and nonlinear frequency conversion, researchers have considered both intracavity oscillation and external cavity spatial modulation. To achieve flexible outputs, spatial light modulators can be used to obtain structured beams both inside and outside the laser cavity. But this is an indirect, inefficient method. Intracavity nonlinear frequency generation of structured beams offers a direct, efficient method that has only rarely been investigated, until recently.
Inside a laser cavity, an effect known as “transverse mode locking” (TML) enables the direct generation of the vortex beams or optical vortices from a laser cavity. It is known that both solid-state microchip lasers and VCSELs can produce quite similar outputs of TML beam patterns under large Fresnel number pumping conditions. The complex transverse patterns formed by the TML effect, commonly composed of different basic modes with different weight coefficients and different locking phases, make for abundant spatial information in fundamental frequency modes. Nonlinear frequency conversion of these directly generated TML beams is of great interest, but not yet well studied.

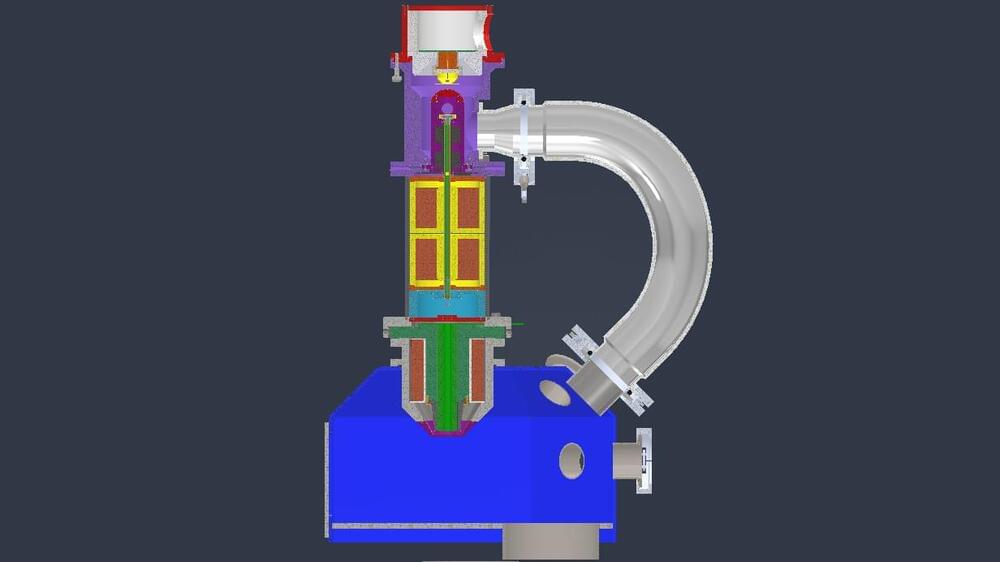
Home Made Scanning Electron Microscope Shows Some Potential
Scanning electron microscopes are one of those niche instruments that most of us don’t really need all the time, but would still love to have access to once in a while. Although we’ve covered a few attempts at home-builds before, many have faltered, except this project over on Hackday. IO by user Vini’s Lab, which appears to be still under active development. The principle of the SEM is pretty simple; a specially prepared sample is bombarded with a focussed beam of electrons, that is steered in a raster pattern. A signal is acquired, using one of a number of techniques, such as secondary electronics (SE) back-scattered electrons (BSE) or simply the transmitted current into the sample. This signal can then be used to form an image of the sample or gather other properties.
The project is clearly in the early stages, as the author says, it’s a very costly thing to build, but already some of the machined parts are ready for assembly. Work has started on the drive electronics for the condenser stigmata lens. This part of the instrument takes the central part of the rapidly diverging raw electron beam that makes it through the anode, and with a couple of sets of octopole coil sets, and an aperture or two, selects only the central portion of the beam, as well as correcting for any astigmatism in the beam. By adjusting the relative currents through each of the coils, a quadrupole magnetic field is created, which counteracts the beam asymmetry.
Scanning control and signal acquisition are handled by a single dedicated card, which utilises the PIO function of a Raspberry Pi Pico module. The Pico can drive the scanning operation, and with an external FTDI USB3.0 device, send four synchronised channels of acquired sample data back to the host computer. Using PCIe connectors and mating edge connectors on the cards, gives a robust and cost effective physical connection. As can be seen from the project page, a lot of mechanical design is complete, and machining has started, so this is a project to keep an eye on in the coming months, and possibly years!