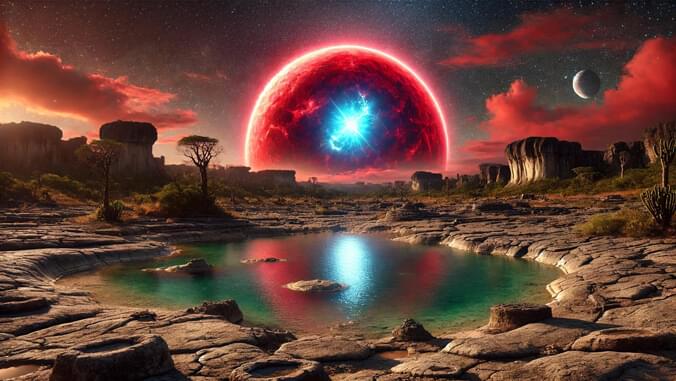
A groundbreaking study has revealed that red dwarf stars can produce stellar flares that carry far-ultraviolet (far-UV) radiation levels much higher than previously believed. This discovery suggests that the intense UV radiation from these flares could significantly impact whether planets around red dwarf stars can be habitable. Led by current and former astronomers from the University of Hawaii Institute for Astronomy (IfA), the research was recently published in the Monthly Notices of the Royal Astronomical Society.
“Few stars have been thought to generate enough UV radiation through flares to impact planet habitability. Our findings show that many more stars may have this capability,” said astronomer Vera Berger, who undertook the study while in the Research Experiences for Undergraduates program at IfA, an initiative supported by the National Science Foundation.
Berger and her team used archival data from the GALEX space telescope to search for flares among 300,000 nearby stars. GALEX is a now-decommissioned NASA mission that simultaneously observed most of the sky at near-and far-UV wavelengths from 2003 to 2013. Using new computational techniques, the team mined novel insights from the data.