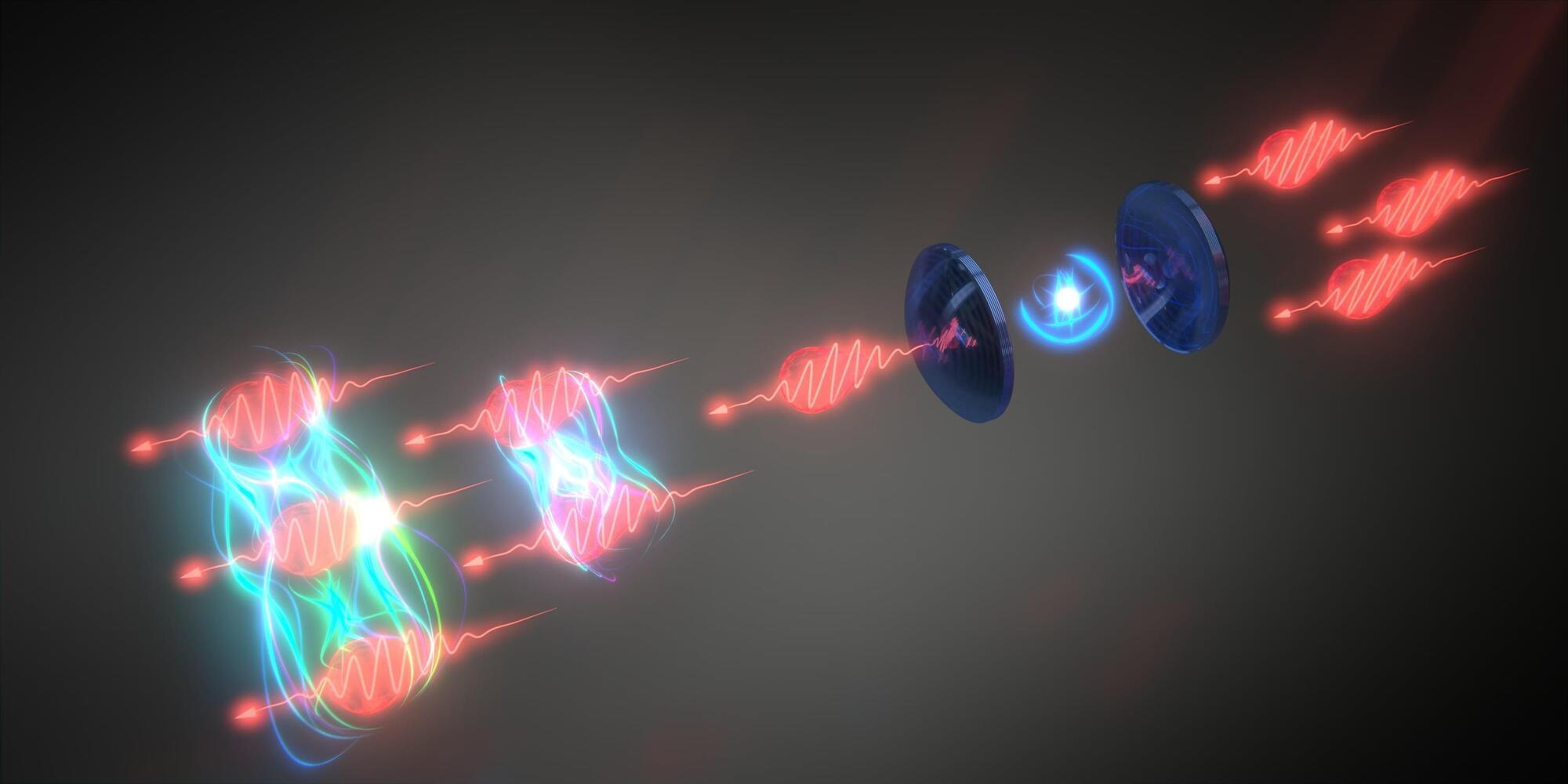
This quantum light manipulation breakthrough paves the way for unprecedented technologies.
Scientists from the University of Basel and the University of Sydney successfully manipulated and identified interacting packets of light energy, or photons, with unprecedented precision.
This breakthrough, published in Nature Physics, marks the first-ever observation of stimulated light emission at the single-photon level—a phenomenon first predicted by Albert Einstein in 1916.
By measuring the time delay between photon interactions, researchers demonstrated how photons could become entangled in a “two-photon bound state,” opening up new possibilities for quantum computing and enhanced measurement techniques.
This discovery has profound implications for photonic quantum computing and metrology, particularly in fields like biological microscopy, where high-intensity light can damage delicate samples. Dr. Sahand Mahmoodian, a leading researcher on the project, emphasized that harnessing quantum light could lead to more precise measurements with fewer photons. Meanwhile, tech companies like PsiQuantum and Xanadu are already exploring how this research could contribute to fault-tolerant quantum computing. As scientists refine their ability to manipulate quantum light, the door opens to a future of more powerful computing, ultra-sensitive sensors, and revolutionary advancements in technology.
Learn more.