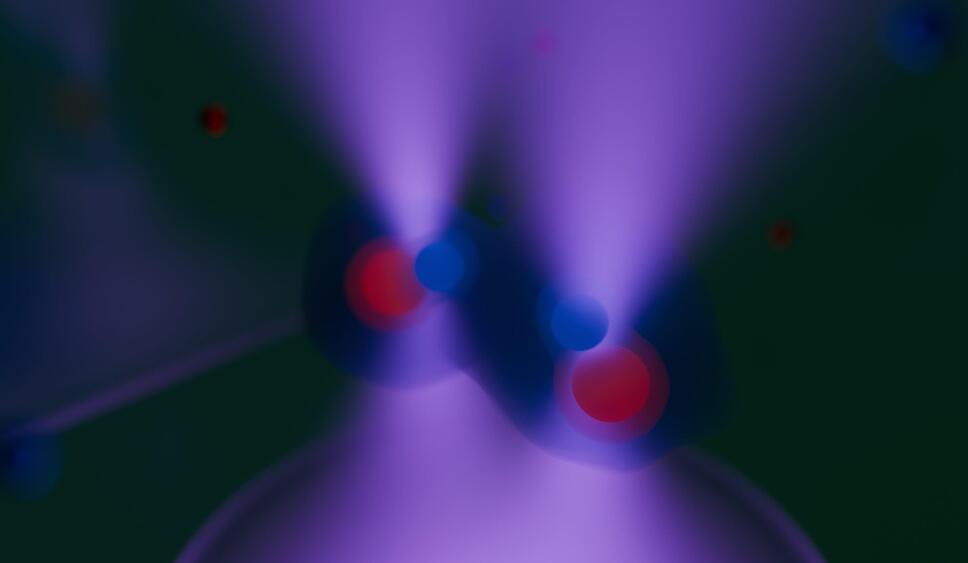
Harnessing molecular connections: unlocking long-lasting quantum entanglement.
Quantum entanglement—the mysterious connection that links particles no matter the distance between them—is a cornerstone for developing advanced technologies like quantum computing and precision measurement tools. While significant strides have been made in controlling simpler particles such as atoms, extending this control to more complex systems like molecules has remained challenging due to their intricate structures and sensitivity to their surroundings.
In a groundbreaking study, researchers have achieved long-lived quantum entanglement between pairs of ultracold polar molecules using a highly controlled environment known as “magic-wavelength optical tweezers.” These tweezers manipulate molecules with extraordinary precision, stabilizing their complex internal states, such as vibrations and rotations, while enabling detectable, fine-scale interactions.
The team successfully created a “Bell state,” a hallmark of quantum entanglement, with pairs of molecules. While some minor errors reduced the initial fidelity of the entangled state, correcting for these issues revealed that the entanglement could persist for remarkably long times—measured in seconds. This is a significant achievement, as second-scale lifetimes are exceptional in the quantum realm.
This breakthrough has far-reaching implications. Long-lived molecular entanglement could enhance quantum sensing technologies, provide new avenues for exploring chemical reactions at ultracold temperatures, and expand the potential of molecules as quantum bits (qubits) in simulations and memory storage for quantum computing. By unlocking the ability to precisely control and entangle molecules, scientists are paving the way for novel applications across quantum science, leveraging the rich internal dynamics of molecular systems.