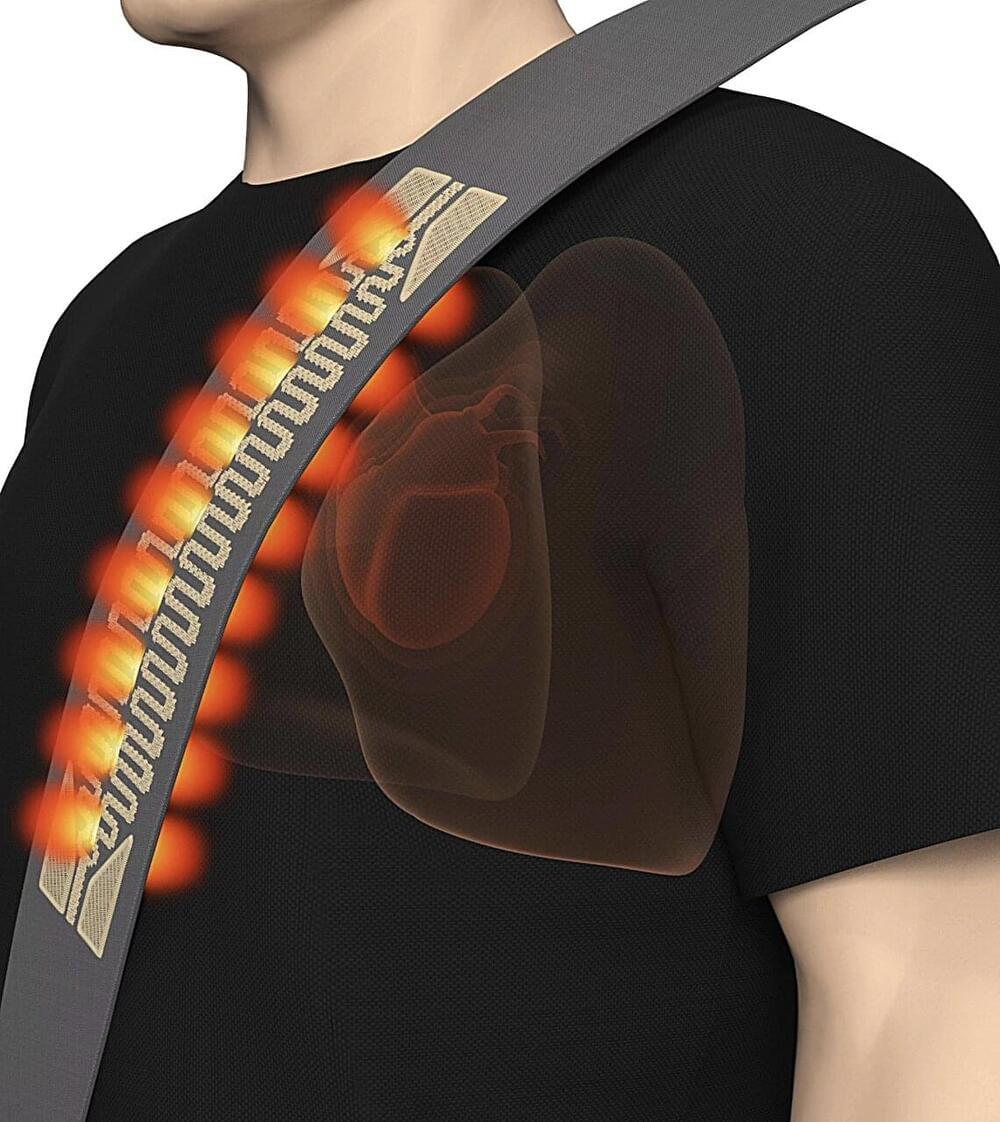
Over the past decades, electronics and biomedical engineers have developed increasingly sophisticated biosensors, devices that can pick up biological signals from human users. These sensors, which are generally embedded in wearable or implantable technologies, often do not perform as well in settings where users are moving a lot, such as within a vehicle.
Researchers at the National University of Singapore and Tsinghua University have recently developed a new sensor that can pick up and track biological signals, such as the heartbeat and respiration, without being in contact with the body of users. This sensor, presented in a paper published in Nature Electronics, could be used to pick up the cardiopulmonary signals of humans while they are in dynamic and closed environments, such as a plane cabin, a moving car or a bus.
“Monitoring drivers’ alertness or stress is essential for road safety,” Xi Tian, co-author of the paper, told Tech Xplore. “Existing sensors designed to measure physiological markers of fatigue, such as heart rate and respiration, face challenges in moving vehicles due to the unpredictable vibrational noise. To overcome these challenges, our research focused on developing an automotive biosensor capable of non-contact and reliable health monitoring in dynamic environments.”