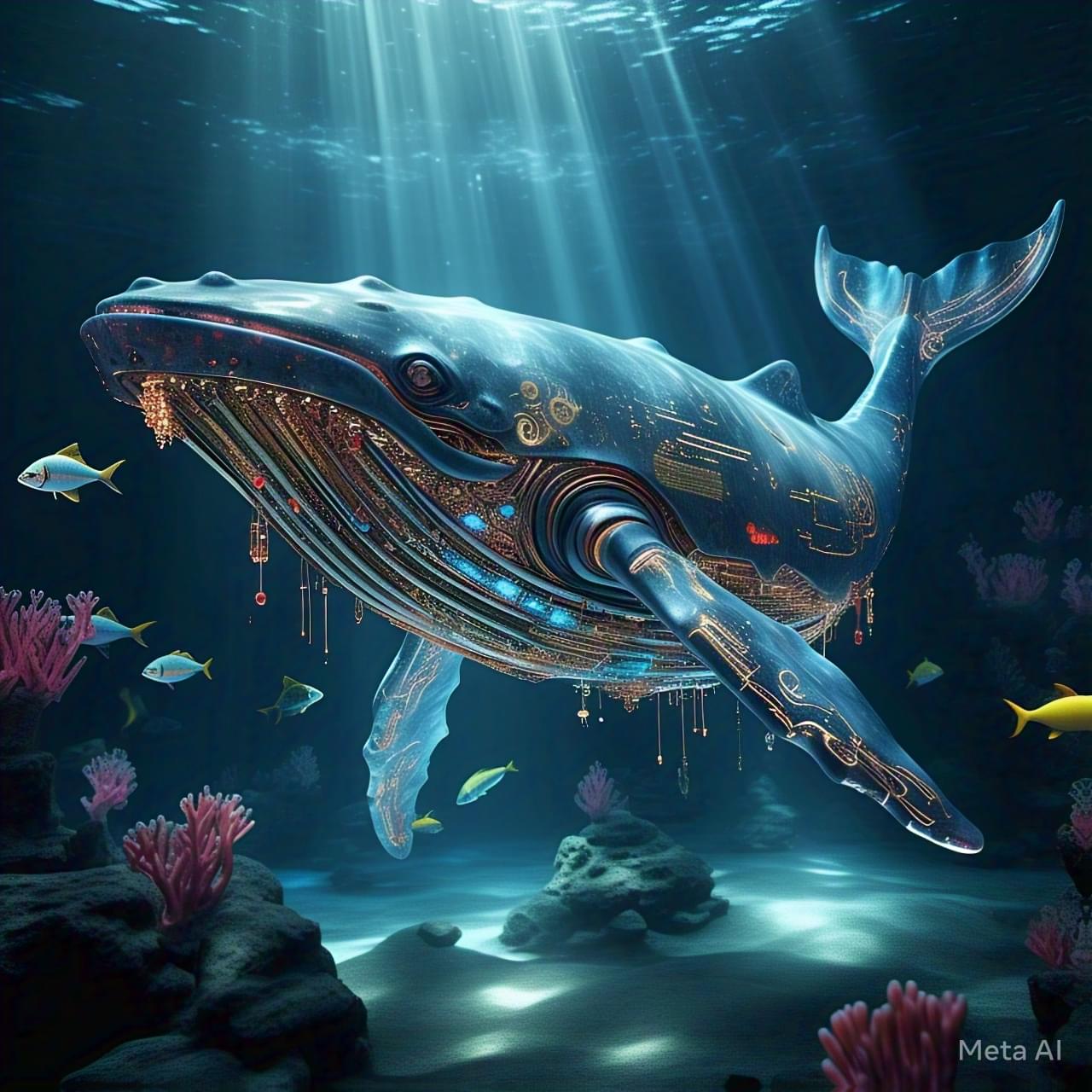
In today’s AI news, Chinese AI start-up DeepSeek wrapped up a week of revealing technical details about its development of a ChatGPT competitor, which was achieved at a fraction of the typical costs, in a move that is poised to accelerate global advances in the field. Over the past few days, DeepSeek published eight open-source projects on GitHub, the world’s largest open-source community.
In other advances, TikTok is preparing to sunset its creator marketplace in favor of a new, more expanded experience, the company has informed businesses and creators via email. The online platform, which connects brands with creators for collaborating on ads and other sponsorships, will stop allowing creator invitations or the creation of new campaigns as of Saturday the company says.
Ll need a Mac with an M1 chip or higher, which means Intel-based Macs are out of the loop. + And, Hume AI has unveiled Octave, an innovative text-to-speech (TTS) system that leverages large language model (LLM) technology to generate contextually aware and emotionally nuanced speech. The incredibly human-like voice tool competitively positions Octave as a leader in AI-driven voice synthesis. Traditional TTS systems often produce context-insensitive speech, which leads to monotonous output.
In videos, Anthropic’s CEO, Dario Amodei, returns to the Hard Fork podcast for a candid, wide-ranging interview. We discuss Anthropic’s brand-new Claude 3.7 Sonnet model, the A.I. arms race against China, and his hopes and fears for this technology over the next two years. Then, we gather up recent tech stories, put them into a hat and close out the week with a round of HatGPT.
And, at the 2025 MIT Bangkok Symposium, Startups and VC Lightning Talks session, the early-entrants include Yunus Sevimli (Almond Fintech), Abhi Yadav (iCustomer), Anuj Bhalla (serviceMob), and Korawad Chearavanont (Amity Solutions). Editors note: I met serviceMob at an Imagination In Action event organized by John Werner of Link Ventures at the MIT-CSAIL Stata Center.
Then, Sam Witteveen looks at olmOCR, the OpenOCR system from Allen AI. There is some additional links for more context of this open OCR project. The collaboration, the blog post about the project, and the macOS version of the product.