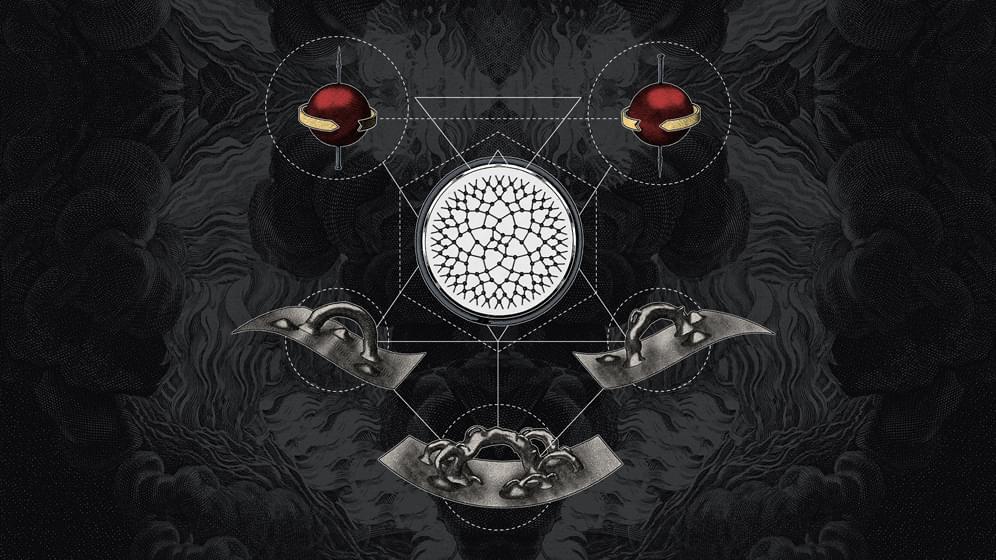
Similarly, entanglement seems to be fundamental to the existence of space-time. This was the conclusion reached by a pair of postdocs in 2006: Shinsei Ryu (now at the University of Illinois, Urbana-Champaign) and Tadashi Takayanagi (now at Kyoto University), who shared the 2015 New Horizons in Physics prize for this work. “The idea was that the way that [the geometry of] space-time is encoded has a lot to do with how the different parts of this memory chip are entangled with each other,” Van Raamsdonk explained.
Inspired by their work, as well as by a subsequent paper of Maldacena’s, in 2010 Van Raamsdonk proposed a thought experiment to demonstrate the critical role of entanglement in the formation of space-time, pondering what would happen if one cut the memory chip in two and then removed the entanglement between qubits in opposite halves. He found that space-time begins to tear itself apart, in much the same way that stretching a wad of gum by both ends yields a pinched-looking point in the center as the two halves move farther apart. Continuing to split that memory chip into smaller and smaller pieces unravels space-time until only tiny individual fragments remain that have no connection to one another. “If you take away the entanglement, your space-time just falls apart,” said Van Raamsdonk. Similarly, “if you wanted to build up a space-time, you’d want to start entangling [qubits] together in particular ways.”
Combine those insights with Swingle’s work connecting the entangled structure of space-time and the holographic principle to tensor networks, and another crucial piece of the puzzle snaps into place. Curved space-times emerge quite naturally from entanglement in tensor networks via holography. “Space-time is a geometrical representation of this quantum information,” said Van Raamsdonk.