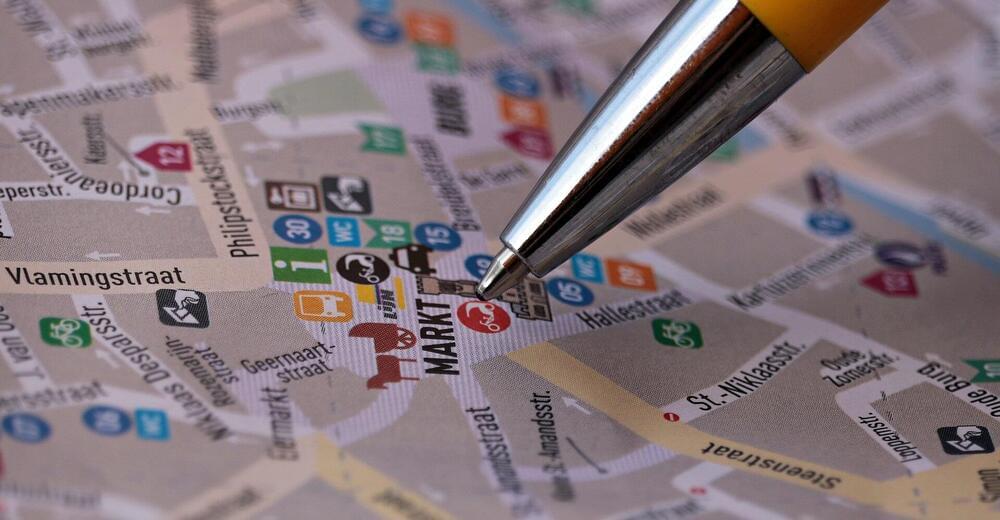
Cities around the globe are experiencing increased flooding due to the compounding effects of stronger storms in a warming climate and urban growth. New research from the University of California, Irvine suggests that urban form, specifically the building density and street network of a neighborhood, is also affecting the intensity of flooding.
For a paper published today in Nature Communications, researchers in UC Irvine’s Department of Civil and Environmental Engineering turned to statistical mechanics to generate a new formula allowing urban planners to more easily assess flood risks presented by land development changes.
Co-author Mohammad Javad Abdolhosseini Qomi, UC Irvine associate professor of civil and environmental engineering who holds a joint appointment in UC Irvine’s Department of Materials Science and Engineering, said that he and his colleagues were inspired by how physicists study intricate systems such as disordered porous solids, glasses and complex fluids to develop universal theories that can explain city-to-city variations in flood hazards.