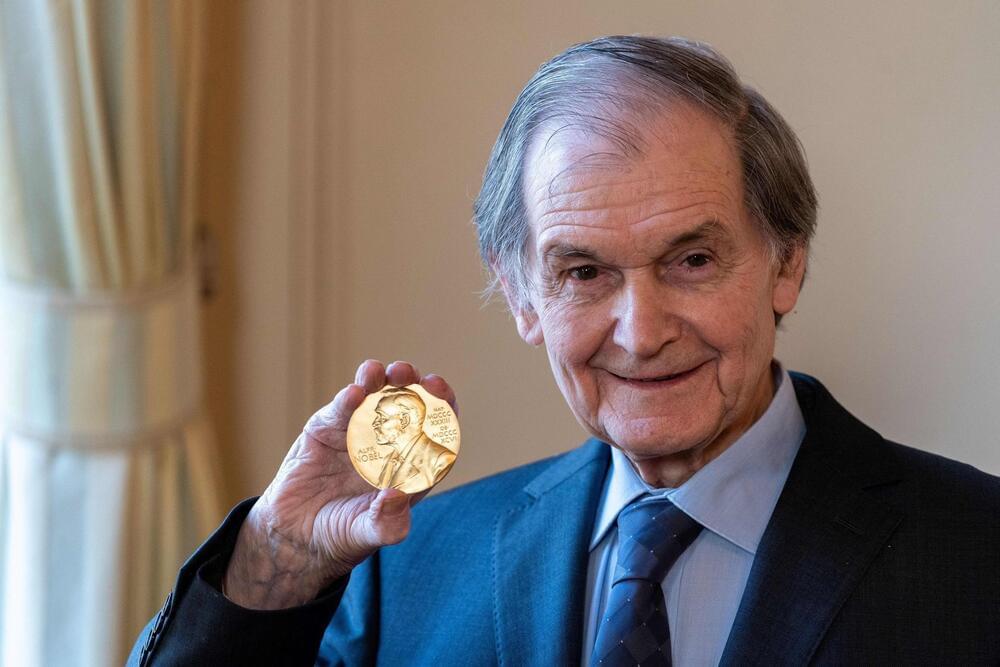
When scientists measure a particle, it seems to collapse to one fixed state. Yet no one can be sure what’s causing collapse, also called reduction of the state. Some scientists and philosophers even think that wave function collapse is an elaborate illusion. This debate is called the measurement problem in quantum mechanics.
The measurement problem has led many physicists and philosophers to believe that a conscious observer is somehow acting on quantum particles. One proposal is that a conscious observer causes collapse. Another theory is that a conscious observer causes the universe to split apart, spiralling out alternate realities. These worlds would be parallel yet inaccessible to us so that we only ever see things in one single state in whatever possible world we’re stuck in. This is the Multiverse or Many Worlds theory. “The point of view that it is consciousness that reduces the state is really an absurdity,” says Penrose, adding that a belief in Many Worlds is a phase that every physicist, including himself, eventually outgrows. “I shouldn’t be so blunt because very distinguished people seem to have taken that view.” Penrose demurs. He politely but unequivocally waves off the idea that a conscious observer collapses wave functions by looking at them. Likewise, he dismisses the view that a conscious observer spins off near infinite universes with a glance. “That’s making consciousness do the job of collapsing the wave function without having a theory of consciousness,” says Penrose. “I’m turning it around and I’m saying whatever consciousness is, for quite different reasons, I think it does depend on the collapse of the wave function. On that physical process.”
What’s causing collapse? “It’s an objective phenomenon,” insists Penrose. He’s convinced this objective phenomenon has to be the fundamental force: gravity. Gravity is a central player in all of classical physics conspicuously missing from quantum mechanics.