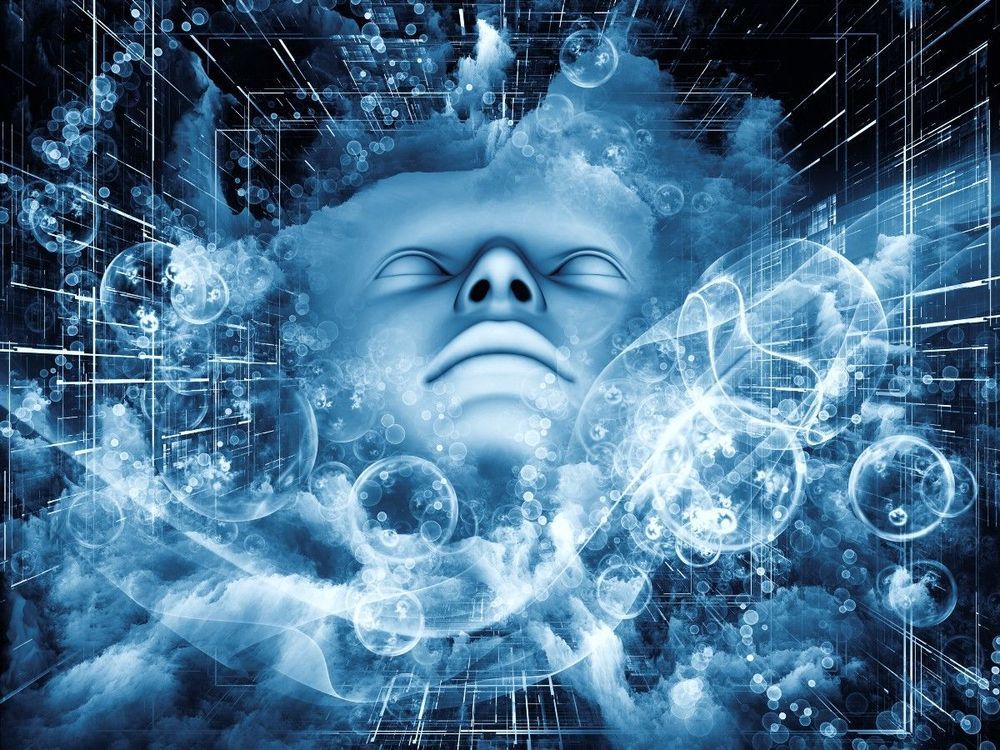
The Universe or any other phenomenon or entity contained therein is not objectively real but subjectively real. Patterns of information emerging from the ultimate code are what is more fundamental than particles of matter or space-time continuum itself all of which is levels below the Code. Nature behaves quantum code-theoretically at all levels. It’s hierarchies of quantum networks all the way down and all the way up. Being part of hierarchical quantum neural networks, a conscious observer system possesses a strange quality: collapsing quantum states of entangled conscious entities and having a privileged interpretation of that. From this perspective, entangled conscious agents would be a mirror conscious environment, whereas the quantum observer would be a central node of the entangled network.
“If we accept that the material universe as we know it is not a mechanical system but a virtual reality created by Absolute Consciousness through an infinitely complex orchestration of experiences, what are the practical consequences of this insight?” –Stanislav Grof
Just like absolute idealism, solipsism certainly defies our common sense but the deeper layer of truth is not what first meets the eye. Here’s what Richard Conn Henry and Stephen Palmquist write in their paper “An Experimental Test of Non-local Realism” (2007): “Why do people cling with such ferocity to belief in a mind-independent reality? It is surely because if there is no such reality (as far as we can know) mind alone exists. And if mind is not a product of real matter, but rather is the creator of the illusion of material reality (which has, in fact, despite the materialists, been known to be the case, since the discovery of quantum mechanics in 1925), then a theistic view of our existence becomes the only rational alternative to solipsism.” One can extend their line of reasoning by arriving at pantheistic solipsism as a likely revelation to ponder about.
Our minds operate in the domains of subjectivity, intersubjectivity and supersubjectivity. In the domain of intersubjectivity, minds create a reality by sharing “mindspace,” i.e. shared belief systems and ways of communication, minds then inhabit the reality which they have created. At the level of your individual mind, i.e. local consciousness, you play a multi-level virtual reality game of life but we all invariably converge at the Omega Singularity by forging our own discrete pathways to the ultimate divine. As you’re reading this right now, you’re now in your own subjective reality tunnel leading to the Source and back where you’re now all of which is definable as a parallel evolutionary feedback process within non-local holistic consciousness patterning this virtual multiverse.