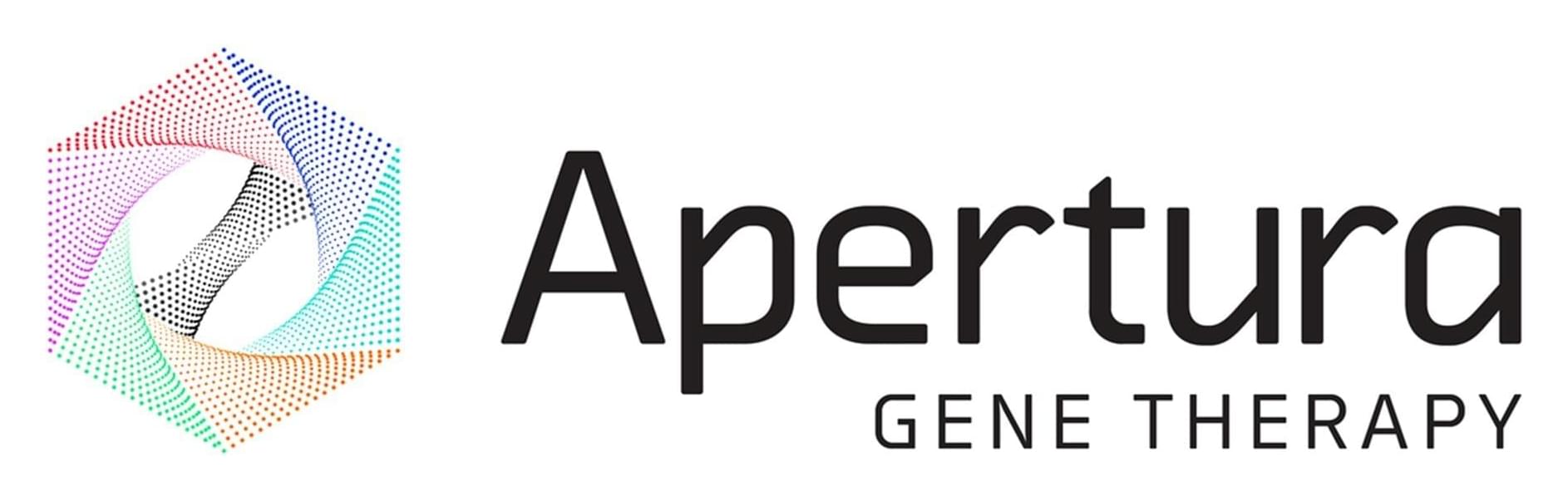
Two remarkable innovations coming together to tackle prion disease: AAVs that leverage human receptors to cross the blood-brain-barrier + a way of epigenetically silencing the gene encoding prions. I recall reading those cited papers and both are amazing!
BOSTON and NEW YORK, Feb. 28, 2025 /PRNewswire/ — Apertura Gene Therapy, a biotechnology company focused on innovative gene therapy solutions, supports the Broad Institute of MIT and Harvard, and the Whitehead Institute in advancing a gene therapy approach for the treatment of prion disease. The project is led by the Vallabh-Minikel lab at the Broad Institute which is focused on finding a cure for prion disease, and their approach leverages two cutting-edge technologies developed at the Institutes of both the Broad and Whitehead: the CHARM platform designed in Dr. Jonathan Weismann’s lab, and TfR1 capsid, an engineered AAV designed in the lab of Dr. Ben Deverman, Director of Vector Engineering at the Broad Institute and scientific founder of Apertura.
Prion disease is a rare, fatal, neurodegenerative disorder caused by misfolded proteins. The new gene therapy aims to address the root cause by using CHARM (Coupled Histone tail for Autoinhibition Release of Methyltransferase) to target and silence the gene that codes for the disease-causing protein1. This payload will be combined with Apertura’s TfR1 capsid, an adeno-associated virus (AAV) capsid engineered to efficiently cross the blood-brain barrier by binding to the human TfR1 receptor, which facilitates iron transport into brain cells2. Together, these technologies represent a transformative approach to tackling CNS diseases.
“We are thrilled to see the progress being made in the development of this innovative therapy for prion disease,” said Dr. Sonia Vallabh, co-leader of the group at the Broad working on preventative therapies for prion disease. “The collaborative efforts between Apertura, the Broad Institute and the Whitehead mark a significant milestone toward addressing unmet needs in neurodegenerative disorders.”