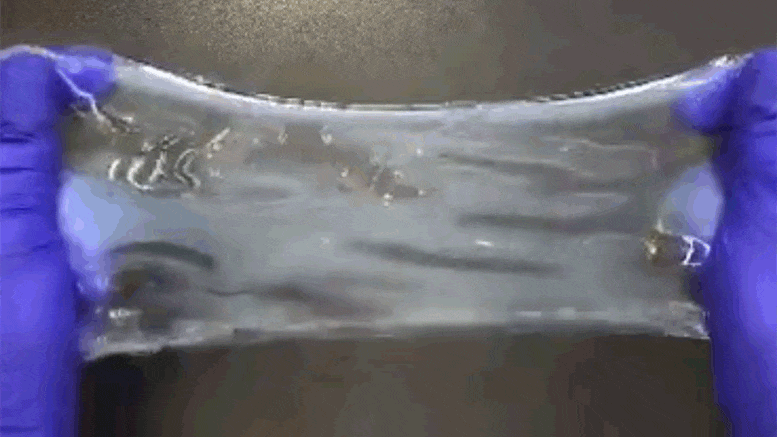
Chemical process called ELAST allows labeling probes to infuse more quickly, and makes samples tough enough for repeated handling.
When there’s a vexing problem to be solved, people sometimes offer metaphorical advice such as “stretching the mind” or engaging in “flexible” thinking, but in confronting a problem facing many biomedical research labs, a team of MIT researchers has engineered a solution that is much more literal. To make imaging cells and molecules in brain and other large tissues easier while also making samples tough enough for years of handling in the lab, they have come up with a chemical process that makes tissue stretchable, compressible, and pretty much indestructible.
“ELAST” technology, described in a new paper in Nature Methods, provides scientists a very fast way to fluorescently label cells, proteins, genetic material, and other molecules within brains, kidneys, lungs, hearts, and other organs. That’s because when such tissues can be stretched out or squished down thin, labeling probes can infuse them far more rapidly. Several demonstrations in the paper show that even after repeated expansions or compressions to speed up labeling, tissues snap back to their original form unaltered except for the new labels.