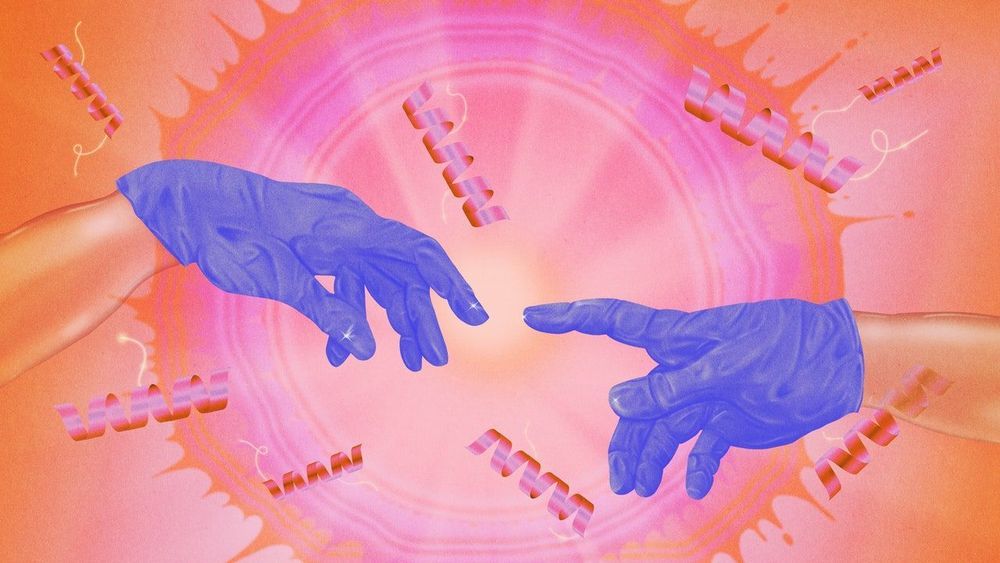
CIEQSFTTLFACQTAAEIWRAFGYTVKIMVDNGNCRLHVC: these forty letters are a set of instructions for building a sophisticated medical device designed to recognize the flu virus in your body. The device latches onto the virus and deactivates the part of it that breaks into your cells. It is impossibly tiny—smaller than the virus on which it operates—and it can be manufactured, in tremendous quantities, by your own cells. It’s a protein.
Proteins—molecular machines capable of building, transforming, and interacting with other molecules—do most of the work of life. Antibodies, which defend our cells against invaders, are proteins. So are hormones, which deliver messages within us; enzymes, which carry out the chemical reactions we need to generate energy; and the myosin in our muscles, which contract when we move. A protein is a large molecule built from smaller molecules called amino acids. Our bodies use twenty amino acids to create proteins; our cells chain them together, following instructions in our DNA. (Each letter in a protein’s formula represents an amino acid: the first two in the flu-targeting protein above are cysteine and isoleucine.) After they’re assembled, these long chains crumple up into what often look like random globs. But the seeming chaos in their collapse is actually highly choreographed. Identical strings of amino acids almost always “fold” into identical three-dimensional shapes. This reliability allows each cell to create, on demand, its own suite of purpose-built biological tools. “Proteins are the most sophisticated molecules in the known universe,” Neil King, a biochemist at the University of Washington’s Institute for Protein Design (I.P.D.), told me. In their efficiency, refinement, and subtlety, they surpass pretty much anything that human beings can build.
Today, biochemists engineer proteins to fight infections, produce biofuels, and improve food stability. Usually, they tweak formulas that nature has already discovered, often by evolving new versions of naturally occurring proteins in their labs. But “de novo” protein design—design from scratch—has been “the holy grail of protein science for many decades,” Sarel Fleishman, a biochemist at the Weizmann Institute of Science, in Israel, told me. Designer proteins could help us cure diseases; build new kinds of materials and electronics; clean up the environment; create and transform life itself. In 2018, Frances Arnold, a chemical engineer at the California Institute of Technology, shared the Nobel Prize in Chemistry for her work on protein design. In April, when the coronavirus pandemic was peaking on the coasts, we spoke over video chat. Arnold, framed by palm trees, sat outside her home, in sunny Southern California. I asked how she thought about the potential of protein design. “Well, I think you just have to look at the world behind me, right?” she said. “Nature, for billions of years, has figured out how to extract resources from the environment—sunlight, carbon dioxide—and convert those into remarkable, living, functioning machines. That’s what we want to do—and do it sustainably, right? Do it in a way that life can go on.”