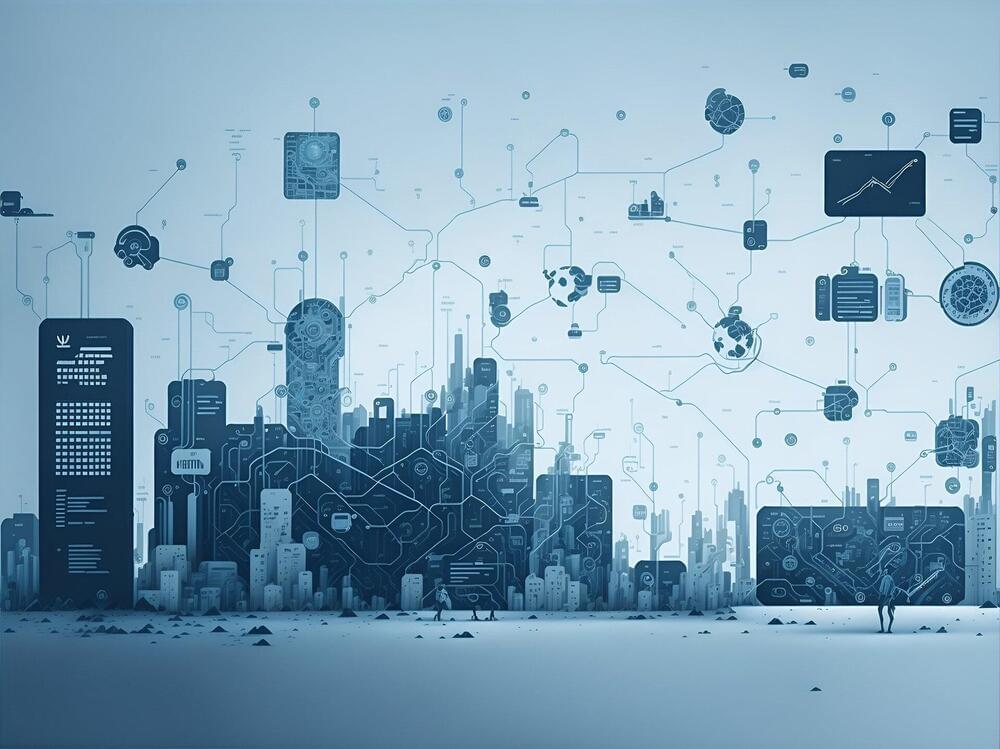
In recent years, artificial intelligence (AI) and deep learning models have advanced rapidly, becoming easily accessible. This has enabled people, even those without specialized expertise, to perform various tasks with AI. Among these models, generative adversarial networks (GANs) stand out for their outstanding performance in generating new data instances with the same characteristics as the training data, making them particularly effective for generating images, music, and text.
GANs consist of two neural networks, namely, a generator that creates new data distributions starting from random noise, and a discriminator which checks whether the generated data distribution is “real” (matching the training data) or “fake.” As training progresses, the generator improves at generating realistic distributions, and the discriminator at identifying the generated data as fake.
GANs use a loss function to measure differences between the fake and real distributions. However, this approach can cause issues like gradient vanishing and unstable learning, directly impacting stability and efficiency. Despite considerable progress in improving GANs, including structural modifications and loss function adjustments, challenges such as gradient vanishing and mode collapse, where the generator produces a limited variety, continue to limit their applicability.