A research team has proposed a wind sensing lidar theory based on up-conversion quantum interference and successfully developed a prototype. Their work is published in ACS Photonics.
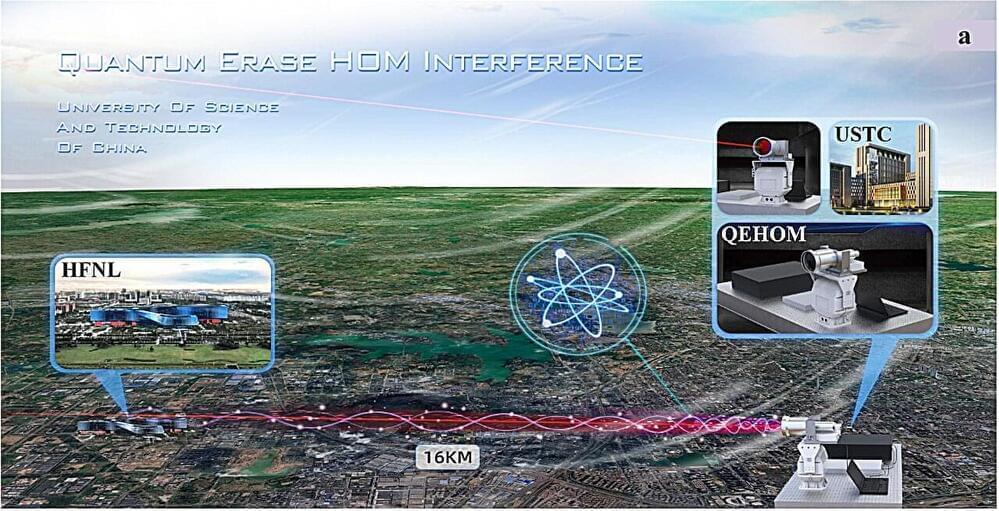
A new study has uncovered the universal dynamics far from equilibrium in randomly interacting spin models, thereby complementing the well-established universality in low-energy equilibrium physics. The study, recently published in Nature Physics, was the result of a collaborative effort involving the research group led by Prof. Du Jiangfeng and Prof. Peng Xinhua at the University of Science and Technology of China (USTC), along with the theoretical groups of Prof. Zhai Hui from Tsinghua University and Dr. Zhang Pengfei from Fudan University.
In 2019, researchers from the Massachusetts Institute of Technology made headlines when they created the “blackest black” material made from carbon nanotubes —ten times blacker than any material that had been manufactured at that time—a material so black that it had the ability to absorb 99.995% of incident light. Such research in light absorption is not a trivial pursuit or mere aesthetics, there are many technologies that can benefit from maximizing light absorption—for instance, in photovoltaics because of the need to absorb and convert as much light as possible into electricity, or on the interior surface of a light sensor because of the need to minimize unwanted stray light. The physics of light absorption can get quite complex when you get into the details, as what we non-technically consider as “black” is usually not a perfect absorber. Indeed, there are many ways to create something that can absorb some light, but the endeavor gets increasingly more difficult the closer one attempts to achieve 100% absorption.
That takes some serious physics.
Now, physicists in Austria and Israel report in the journal Science that they have engineered a light trap that utilizes the quantum properties of electromagnetic waves— in which waveforms undergo constructive or destructive interference when combined in just the right manner—to generate an anti-laser that has near-perfect light absorption [1]. Because the light trap functions essentially as a time-reversed laser, where instead of multiple passes of single-wavelength light for maximum stimulated emission of photons the multiple passes are engineered for maximum absorption, the device is a veritable anti-laser.
Fascinating as this may sound, not all dreams serve as gateways to parallel timelines. Whether dreams return is key here. “Recurring dreams, especially those with vivid and consistent scenarios, might suggest deeper connections to other realities,” Leong claims. On the other hand, dreams tied to personal experiences often feel disjointed, with distorted time. The most surreal and incomprehensible dreams are likely the subconscious processing your life here on Earth, he says. But, if it feels like you’re visiting the dream rather than imagining it—like a play with a beginning, middle, and end—you probably are visiting this other world, under Leong’s hypothesis.
Leong also hints that strong emotions in persistent dreams could offer cosmic clues—signals of how another version of you is experiencing life in a parallel world. “Say you have a repetitive dream of being stuck in high school,” he suggests. “While it may reflect unresolved psychological themes, such as feelings of stagnation or anxiety about personal growth, it could also indicate that in another reality, you are still in high school, dealing with the same challenges your waking self has moved beyond.” This emotional resonance—like the frustration of being stuck—could ripple across dimensions, creating a feedback loop between your conscious mind here and one of your alter egos elsewhere.
YET, AS CAPTIVATING AS THIS HYPOTHESIS MIGHT BE, it runs into a significant problem: there’s no empirical evidence to back it up. Quantum phenomena, such as entanglement and nonlocality, challenge our traditional views on time and space. Yet, no scientific studies conclusively support the idea that dreams are portals to other worlds. Mainstream neuroscience and cognitive science, on the other hand, find this hypothesis heretical—if not downright unscientific.
In a world powered by artificial intelligence applications, data is king, but it’s also the crown’s biggest burden.
As described in the article, quantum memory stores data in ways that classical memory systems cannot match. In quantum systems, information is stored in quantum states, using the principles of superposition and entanglement to represent data more efficiently. This ability allows quantum systems to process and store vastly more information, potentially impacting data-heavy industries like AI.
In a 2021 study from the California Institute of Technology, researchers showed that quantum memory could dramatically reduce the number of steps needed to model complex systems. Their method proved that quantum algorithms using memory could require exponentially fewer steps, cutting down on both time and energy. However, this early work required vast amounts of quantum memory—an obstacle that could have limited its practical application.
Now, two independent teams have derived additional insights, demonstrating how these exponential advantages can be achieved with much less quantum memory. Sitan Chen from Harvard University, along with his team, found that just two quantum copies of a system were enough to provide the same computational efficiency previously thought to require many more.
The challenge of observing and controlling complex phases of matter has long intrigued researchers, particularly when it comes to non-equilibrium systems like time crystals.
Researchers successfully observed topological time-crystalline order using superconducting qubits on a programmable quantum processor.
Predicting the behavior of many interacting quantum particles is a complex task, but it’s essential for unlocking the potential of quantum computing in real-world applications. A team of researchers, led by EPFL, has developed a new method to compare quantum algorithms and identify the most challenging quantum problems to solve.
Quantum systems, from subatomic particles to complex molecules, hold the key to understanding the workings of the universe. However, modeling these systems quickly becomes overwhelming due to their immense complexity. It’s like trying to predict the behavior of a massive crowd where everyone constantly influences everyone else. When you replace the crowd with quantum particles, you encounter what’s known as the “quantum many-body problem.”
Quantum many-body problems involve predicting the behavior of numerous interacting quantum particles. Solving these problems could lead to major breakthroughs in fields like chemistry and materials science, and even accelerate the development of technologies like quantum computers.
Researchers have achieved a breakthrough in observing intrinsic magnetic structures in kagome lattices, which may significantly influence future quantum computing and superconductivity applications.
A research team led by Prof. Qingyou Lu from the Hefei Institutes of Physical Science at the Chinese Academy of Sciences, in collaboration with Prof. Yimin Xiong from Anhui University, has achieved a groundbreaking discovery. Using advanced techniques such as magnetic force microscopy (MFM), electron paramagnetic resonance spectroscopy, and micromagnetic simulations, they have made the first-ever observation of intrinsic magnetic structures within a kagome lattice.
These findings, published recently in Advanced Science, shed new light on the behavior of materials, which is largely determined by the interaction between their internal electrons and lattice structure. Kagome lattices, known for their unique properties like Dirac points and flat bands, display extraordinary phenomena such as topological magnetism and unconventional superconductivity. These lattices are of great interest because of their potential to unlock new insights into high-temperature superconductivity and quantum computing. Despite this, the intrinsic spin patterns that define these materials have remained elusive—until now.
NASA has temporarily halted operations of its quantum computer after it produced unexpected results. The computer, which is still under development, is designed to simulate complex systems such as those found in space. However, during a recent test, the computer-generated results that were inconsistent with known physical laws.
NASA scientists are currently investigating the cause of the anomaly. They are also working to develop safeguards to prevent similar incidents from happening in the future.
The shutdown of the quantum computer is a setback for NASA’s efforts to develop new technologies for space exploration. However, it is also an opportunity to learn more about the potential of quantum computing.