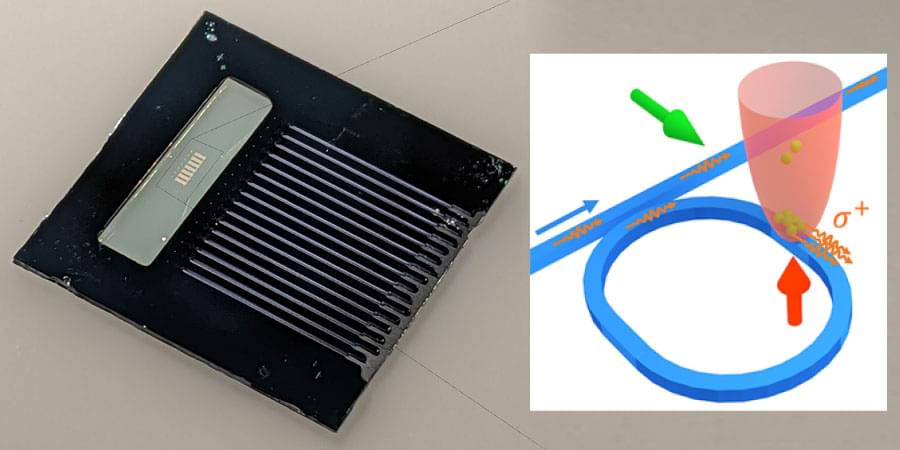
Researchers at Purdue University have trapped alkali atoms (cesium) on an integrated photonic circuit, which behaves like a transistor for photons (the smallest energy unit of light) similar to electronic transistors. These trapped atoms demonstrate the potential to build a quantum network based on cold-atom integrated nanophotonic circuits. The team, led by Chen-Lung Hung, associate professor of physics and astronomy at the Purdue University College of Science, published their discovery in the American Physical Society’s Physical Review X (“Trapped Atoms and Superradiance on an Integrated Nanophotonic Microring Circuit”).
“We developed a technique to use lasers to cool and tightly trap atoms on an integrated nanophotonic circuit, where light propagates in a small photonic ‘wire’ or, more precisely, a waveguide that is more than 200 times thinner than a human hair,” explains Hung, who is also a member of the Purdue Quantum Science and Engineering Institute. “These atoms are ‘frozen’ to negative 459.67 degrees Fahrenheit or merely 0.00002 degrees above the absolute zero temperature and are essentially standing still. At this cold temperature, the atoms can be captured by a ‘tractor beam’ aimed at the photonic waveguide and are placed over it at a distance much shorter than the wavelength of light, around 300 nanometers or roughly the size of a virus. At this distance, the atoms can very efficiently interact with photons confined in the photonic waveguide. Using state-of-the-art nanofabrication instruments in the Birck Nanotechnology Center, we pattern the photonic waveguide in a circular shape at a diameter of around 30 microns (three times smaller than a human hair) to form a so-called microring resonator. Light would circulate within the microring resonator and interact with the trapped atoms.”
A key aspect function the team demonstrates in this research is that this atom-coupled microring resonator serves like a ‘transistor’ for photons. They can use these trapped atoms to gate the flow of light through the circuit. If the atoms are in the correct state, photons can transmit through the circuit. Photons are entirely blocked if the atoms are in another state. The stronger the atoms interact with the photons, the more efficient this gate is.