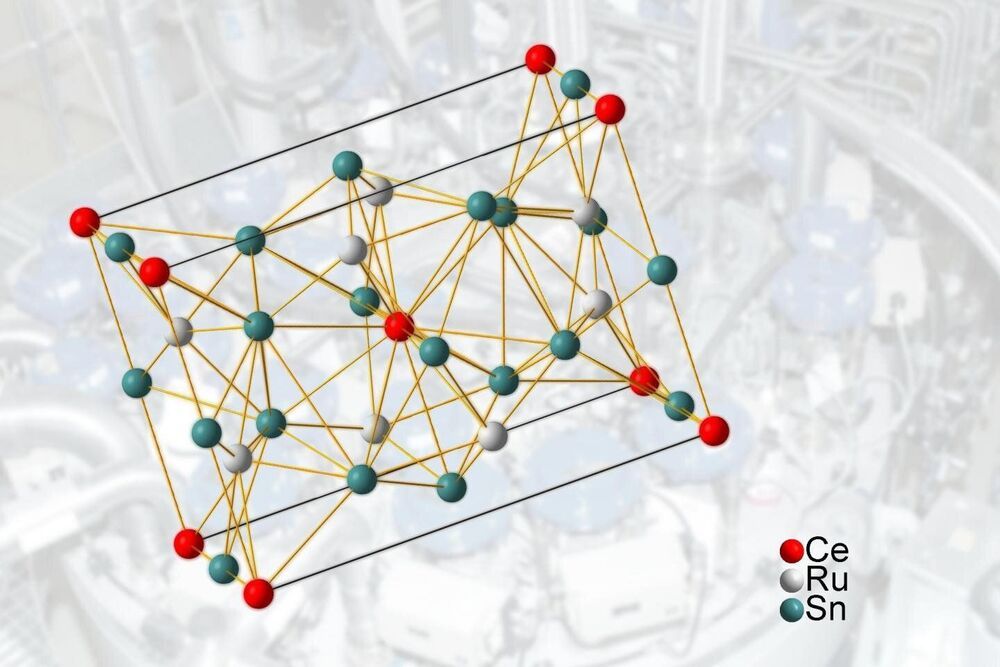
## SCIENCE ADVANCES • MAY 24, 2021 # *by Vienna University of Technology*
In everyday life, phase transitions usually have to do with temperature changes--for example, when an ice cube gets warmer and melts. But there are also different kinds of phase transitions, depending on other parameters such as magnetic field. In order to understand the quantum properties of materials, phase transitions are particularly interesting when they occur directly at the absolute zero point of temperature. These transitions are called "quantum phase transitions" or a "quantum critical points."
Such a quantum critical point has now been discovered by an Austrian-American research team in a novel material, and in an unusually pristine form. The properties of this material are now being further investigated.
It is suspected that the material could be a so-called Weyl-Kondo semimetal, which is considered to have great potential for quantum technology due to special quantum states (so-called topological states). If this proves to be true, a key for the targeted development of topological quantum materials would have been found.
This surprising result is probably related to the fact that the behavior of electrons in this material has some special features. "It is a highly correlated electron system. This means that the electrons interact strongly with each other, and that you cannot explain their behavior by looking at the electrons individually.
If there are only relatively few free electrons, as is the case in a semimetal, then the Kondo effect is unstable. This could be the reason for the quantum critical behavior of the material: the system fluctuates between a state with and a state without the Kondo effect, and this has the effect of a phase transition at zero temperature.
**Quantum fluctuations could lead to Weyl particles**