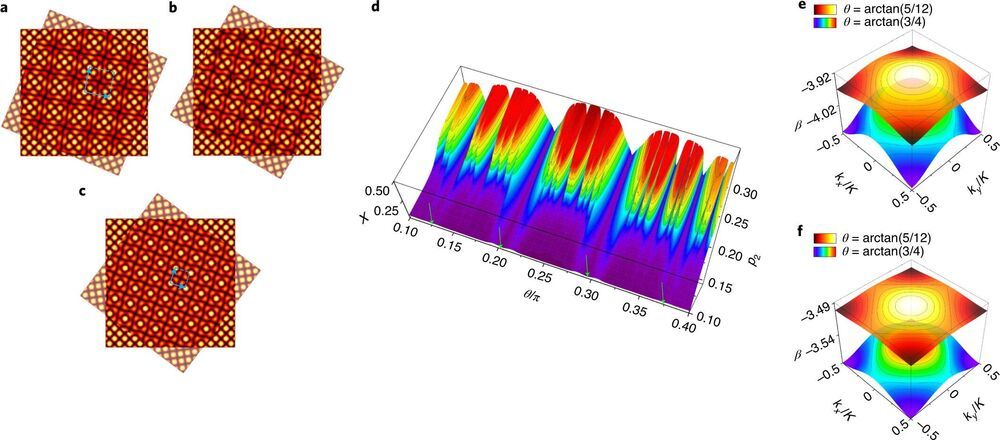
A team of researchers from China, Spain, Russia and Portugal has developed a way to use Moiré lattices to optically induce and highlight the formation of optical solitons under different geometrical conditions. In their paper published in the journal Nature Photonics, the group describes their work, which involved using photorefractive nonlinear media as a means of localizing laser light into tight spots.
Solitons are quasiparticles propagated by a traveling wave. Unlike waves such as those produced in water, solitons are neither followed nor preceded by other such waves—they also hold their shape as they move. They are important because they are able to prevent diffraction from occurring, which is why they play such an important role in telecommunications and other information carrier systems. Moiré lattices are patterns that sometimes emerge in printed or scanned images when two patterns overlap one another in an offset fashion. They have been used in graphene-based research efforts and work that involves manipulating very cold atoms. They have also been found to play a roll in the development of colloidal clusters.
In this new work, the researchers were investigating the ways that light could be stopped from spreading—more specifically, ways that laser light could be trapped in a tight spot. To that end, they used a laser beam to stencil a special a type of crystal: a photorefractive strontium barium niobite crystal with nonlinear holographic properties. The stencil forced a beam of laser light to form into a twisted Moiré lattice. As the light moved through the lattice, the researchers found that solitons formed. They also found that they could adjust the threshold of the laser power by fine-tuning the angles of the twists in the lattice. Additionally, the formation of solitons in the lattices occurred with smooth transitions, from fully periodic geometries to aperiodic ones. The researchers also noted that such thresholds in their setup were quite low.