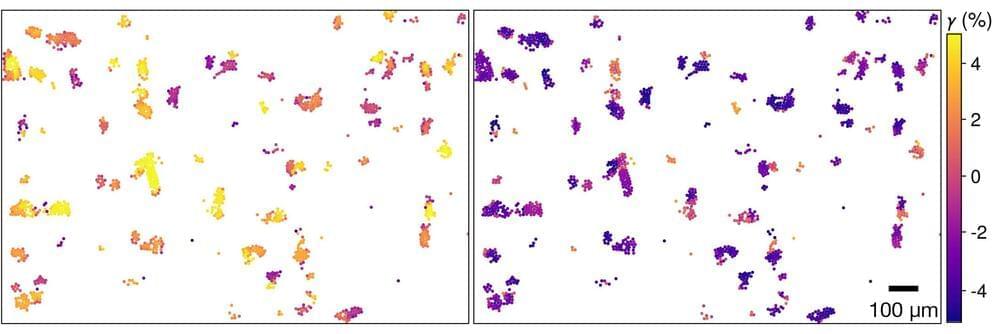
Some solid materials have a memory of how they have previously been stretched out, which impacts how they respond to these kinds of deformations in the future. A new Penn State study lends insight into memory formation in the foams and emulsions common in food products and pharmaceuticals and provides a new method to erase this memory, which could guide how materials are prepared for future use.
“A crease in a piece of paper serves as a memory of being folded or crumpled,” said Nathan Keim, associate research professor of physics at Penn State who led the study. “A lot of other materials form memories when they are deformed, heated up, or cooled down, and you might not know it unless you ask the right questions. Improving our understanding of how to write, read, and erase memories provides new opportunities for diagnostics and programming of materials. We can find out the history of a material by doing some tests or erase a material’s memory and program a new one to prepare it for consumer or industrial use.”
The researchers studied memory in a type of material called disordered solids, which have particles that are often erratically arranged. For example, ice cream is a disordered solid made up of a combination of ice crystals, fat droplets, and air pockets mixed together in a random way. This is in stark contrast to materials with “crystalline structures,” with particles arranged in highly ordered rows and columns. Disordered solids are common in food sciences, consumer products, and pharmaceuticals and include foams like ice cream and emulsions like mayonnaise.