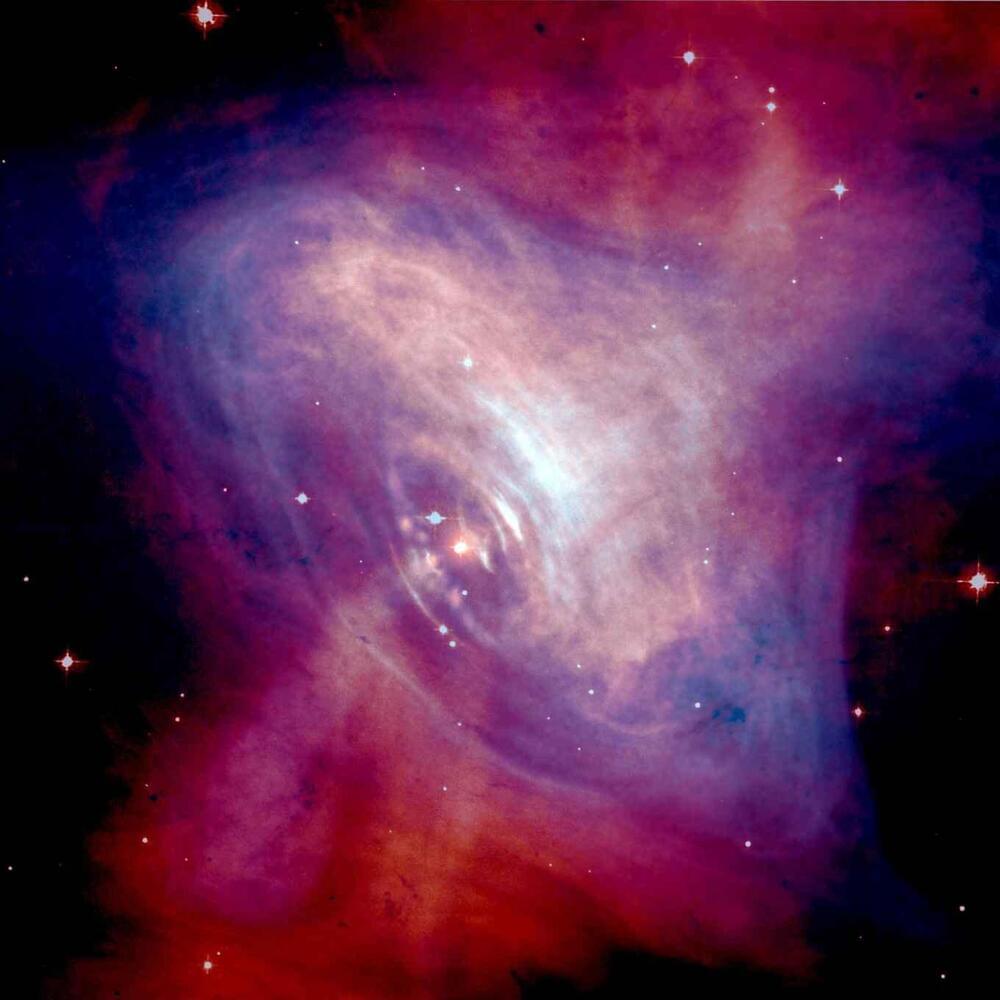
The central question in the ongoing hunt for dark matter is: what is it made of? One possible answer is that dark matter consists of particles known as axions. A team of astrophysicists, led by researchers from the universities of Amsterdam and Princeton, has now shown that if dark matter consists of axions, it may reveal itself in the form of a subtle additional glow coming from pulsating stars. Their work is published in the journal Physical Review Letters.
Dark matter may be the most sought-for constituent of our universe. Surprisingly, this mysterious form of matter, that physicist and astronomers so far have not been able to detect, is assumed to make up an enormous part of what is out there.
No less than 85% of matter in the universe is suspected to be “dark,” presently only noticeable through the gravitational pull it exerts on other astronomical objects. Understandably, scientists want more. They want to really see dark matter—or at the very least, detect its presence directly, not just infer it from gravitational effects. And, of course: they want to know what it is.