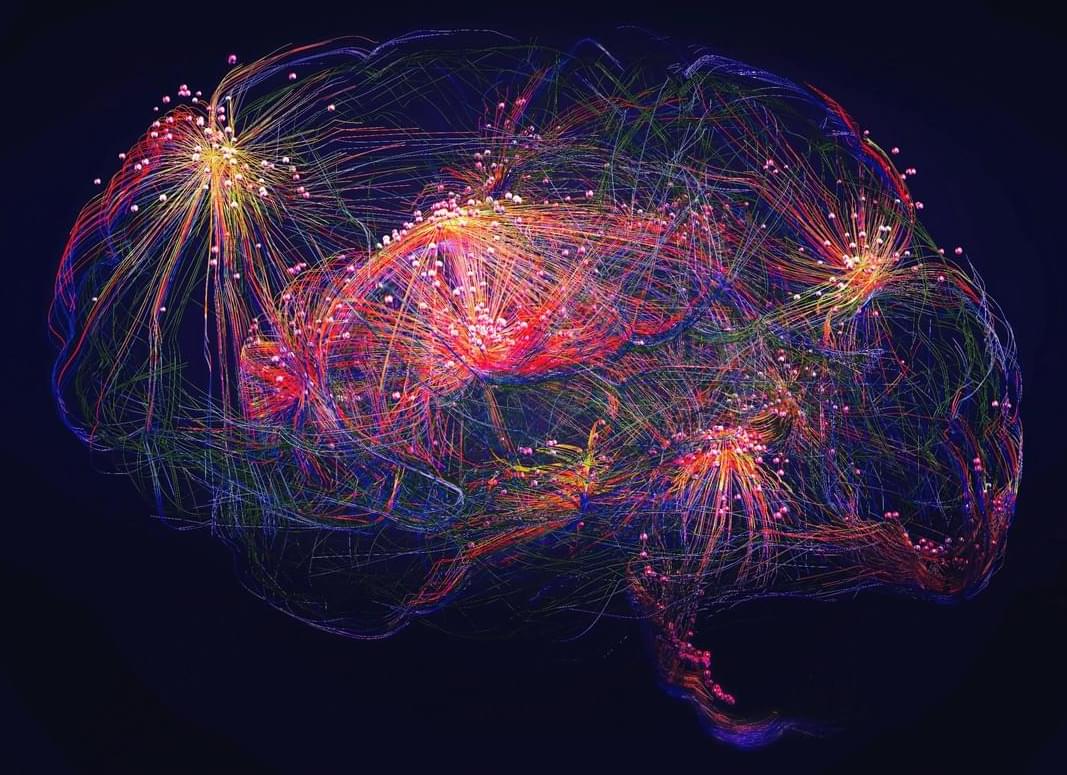
Vertical columns How the Brain Maps Jaw Movements: A Hidden Architecture of Motion.
Our brains contain intricate maps that guide every voluntary movement we make, from reaching out to grab a cup to the delicate motions involved in speaking or chewing. But how exactly are these maps organized, and what role do different types of brain cells play in shaping them?
A new study dives deep into the orofacial motor maps—the brain’s blueprint for controlling jaw movements—revealing a surprising level of organization. Researchers used optogenetics, a technique that activates specific neurons with light, to map out how different classes of excitatory neurons contribute to jaw motion in mice. What they found was remarkable: rather than a single unified map, the motor cortex is divided into distinct, genetically defined modules, each governing jaw movement from different brain regions, including sensory, motor, and premotor areas.
These modules don’t act in isolation. When one was stimulated, activity rippled across the brain, converging in the primary motor cortex, the region that directly controls movement. What’s more, when the mice learned new motor skills—such as refining their licking motion—some of these modules expanded, adapting to support the learned behavior.
This research suggests that voluntary movement isn’t just dictated by a single command center. Instead, a network of specialized cell groups collaborates across different parts of the brain, dynamically adjusting as we learn new motor skills. Understanding this fine-tuned motor map could have implications for treating movement disorders or even advancing brain-computer interfaces in the future.
Scientists have identified previously unknown neural modules in the brain that control movement and adapt during skill learning. Their findings challenge long-held ideas about how the brain organizes movement.