https://www.stitcher.com/podcast/reaching-the-finish-line/e/…oplay=true
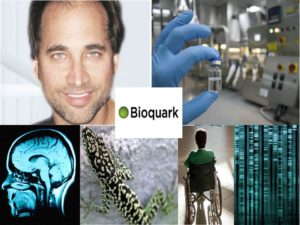
Summary: Researchers at the University of California discovered a key way that cancer manipulates the genetic code using DNA methylation that has important implications for the treatment of cancers. [This article first appeared on the website LongevityFacts.com. Author: Brady Hartman. ]
Up until now, scientists haven’t fully understood how DNA methylation causes changes in our genetic code that enable cancer to thrive.
Now, a team led by associate professor Jikui Song at the University of California Riverside have deciphered the crystal structure of an enzyme that plays a crucial role in DNA methylation that allows tumors to survive and grow.
You can’t peer very far down into a well or below the surface of the ocean before things go dark—light does not penetrate to such depths. Though the brain is far from bottomless, neuroscientists face the same lack of light when they try to study living deep-brain structures. This is especially frustrating given that optogenetics, a method for manipulating genetically tagged brain cells with light, has exploded in popularity over the past decade. “Optogenetics has been a revolutionary tool for controlling neurons in the lab, and hopefully someday in the clinic,” says Thomas McHugh, research group leader at the RIKEN Brain Science Institute in Japan. “Unfortunately, delivering light within brain tissue requires invasive optical fibers.”
McHugh and colleagues now have a solution for sending light to new depths in the brain. As they report in Science on February 9, upconversion nanoparticles (UCNPs) can act as a conduit for laser light delivered from outside the skull. These nanoparticles absorb near-infrared laser light and in turn emit visible photons to areas that are inaccessible to standard optogenetics. This method was used to turn on neurons in various brain areas as well as silence seizure activity and evoke memory cells. “Nanoparticles effectively extend the reach of our lasers, enabling the ‘remote’ delivery of light and potentially leading to non-invasive therapies,” says McHugh.
In optogenetics, blue-green light is used to turn neurons on or off via light-responsive ion channels. Light at these wavelengths, however, scatters strongly and is at the other end of the spectrum from the near-infrared light that can penetrate deeper into brain tissue. UCNPs composed of elements from the lanthanide family can act as a bridge. Their ‘optogenetic actuation’ turns low-energy near-infrared laser light into blue or green wavelengths for control of specifically labeled cells. Though such bursts of light deliver considerable energy to a small area, temperature increases or cellular damage were not observed.
Major psychiatric disorders like autism, schizophrenia and bipolar appear to have more in common than we thought they did. A new study finds that they have important similarities at a molecular level.
And understanding the molecular basis of major disorders such as autism, schizophrenia and bipolar is hopeful, because it could help in developing better treatments for them.
These psychiatric disorders are diagnosed by how a patient behaves. There are no clear signs on a brain “that you can see with your eyes or most microscopic techniques,” says Dan Geschwind, a professor of neurogenetics at UCLA. His team’s findings were published in Science this week.
Summary: Regenerative reprogramming of the body with injections could turn back the clock in our organs, say scientists in a Jan 2018 report. Prematurely aged mice lived 30% longer after Salk Institute researchers genetically reprogrammed their cells while still in the body. [This article first appeared on the website LongevityFacts.com. Author: Brady Hartman. ]
Imagine a time in the future when the doctor gives you the bad news: you need a heart transplant. In the past, this would be an expensive and drastic procedure. Instead, the doctor gives you an injection that rejuvenates your heart. In time, your heart eventually mends itself, reverting to the strength and vigor of your youth.
The dream is closer to reality than you think, thanks to a novel technique developed by a team researchers headed by Juan Carlos Izpisua Belmonte at the Salk Institute in La Jolla.
A new study says that our aging immune system plays a larger role in explaining cancer than genetic mutations. The new findings support the idea of rejuvenating the thymus — an essential immune system organ — as a strategy to prevent cancer.
Summary: A new study says that our aging immune system plays a larger role in explaining cancer than genetic mutations. The new findings support the idea of rejuvenating the thymus – an essential immune system organ – as a strategy to prevent cancer. [This article first appeared on LongevityFacts. Author: Brady Hartman. ]
A new study suggests that our aging immune system plays a larger role in cancer than previously thought, challenging the conventional notion that genetic mutations are the primary driver of tumors.
The research suggests that the key to cancer prevention and treatment may lie in bolstering the immune system, using techniques such as restoring thymus function, rather than addressing genetic mutations. The genetic mutations that occur as the result of DNA damage are the current focus of most anti-cancer efforts throughout the world. These new findings could have significant implications for the fight against cancer if borne out by additional studies.