She confirmed dark matter, probed spiral galaxies and fought inequality.
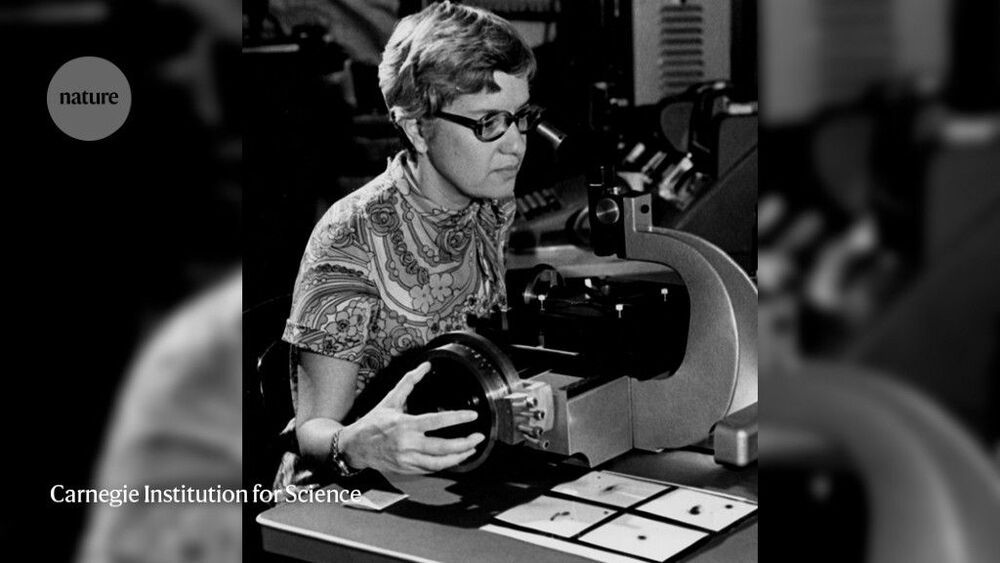
About half of the relatively small portion of the universe that is not dark matter or energy is in fact a mixture of gases that may connect galaxies in a kind of loose cosmic web, according to new research illuminating vast areas that were previously unknown.
Until now, this sizable chunk of “baryonic matter,” which makes up 5% of the universe, had been unaccounted for. Researchers from institutions in Spain and the Argonne National Laboratory in Illinois detailed their findings in a study published March 25 in Monthly Notices of the Royal Astronomical Society.
While the other 95% of the universe is made up of dark matter and dark energy, baryonic matter comprises stars, planets, galaxies and everything they contain, including living things. Astronomers knew it was there, but didn’t know if it was more stars, planets or anything else that wasn’t dark matter or energy.
Great new episode with NASA cosmologist Jason Rhodes who discusses everything from the earliest galaxy surveys to dark matter and the cosmic web.
Jason Rhodes, a cosmologist at NASA’s Jet Propulsion Laboratory in Pasadena, and the JPL Roman Space Telescope Project Scientist, discusses a proposed galaxy survey to end all galaxy surveys. One that would wring as much information out of our universe’s trillion or so galaxies across cosmic time as humanly possible. Astronomers are still at least half a century off from this final galaxy census, but the hope is that it will give cosmologists most of the answers they need about the makeup and structure of the universe.
Researchers using NASA’s upcoming James Webb Space Telescope will map and model the core of nearby galaxy Centaurus A.
As technology has improved over the centuries, so have astronomers’ observations of nearby galaxy Centaurus A. They have peeled back its layers like an onion to discover that its wobbly shape is the result of two galaxies that merged more than 100 million years ago. It also has an active supermassive black hole, known as an active galactic nucleus, at its heart that periodically sends out twin jets. Despite these advancements, Centaurus A’s dusty core is still quite mysterious. Webb’s high-resolution infrared data will allow a research team to very precisely reveal all that lies at the center.
“Whooshes of creation” may be producing multiverses at this moment, says astronomer.
The cosmic inflation credited with creating the homogeneous universe which we now enjoy was likely not a one-off event, University of California, Berkeley astronomer Alexei Filippenko, told me. In fact, these ‘whooshes of creation’ may be producing multiverses even at this moment, says Filippenko.
The idea of an exponential, faster-than-light expansion of the early universe, was first put forth by MIT astrophysicist Alan Guth in 1981. And today, Inflation theory is used to explain the Cosmos’ current size, expansion, homogeneity and the fact that it appears to be geometrically flat, I noted in a 2011 issue of Astronomy magazine.
“Inflation happened within the first trillionth of a trillionth of a trillionth of a second of the universe’s existence,” said Filippenko. “It disappeared and then transformed itself into the material that eventually formed us.”
Our sky is missing supernovas. Stars live for millions or billions of years. But given the sheer number of stars in the Milky Way, we should still expect these cataclysmic stellar deaths every 30–50 years. Few of those explosions will be within naked-eye-range of Earth. Nova is from the Latin meaning “new”. Over the last 2000 years, humans have seen about seven “new” stars appear in the sky – some bright enough to be seen during the day – until they faded after the initial explosion. While we haven’t seen a new star appear in the sky for over 400 years, we can see the aftermath with telescopes – supernova remnants (SNRs) – the hot expanding gases of stellar explosions. SNRs are visible up to a 150000 years before fading into the Galaxy. So, doing the math, there should be about 1200 visible SNRs in our sky but we’ve only managed to find about 300. That was until “Hoinga” was recently discovered. Named after the hometown of first author Scientist Werner Becker, whose research team found the SNR using the eROSITA All-Sky X-ray survey, Hoinga is one of the largest SNRs ever seen.
Hoinga is big. Really big. The SNR spans 4 degrees of the sky – eight times wider than the Full Moon. The obvious question – how could astronomers not have already found something THAT enormous? Hoinga is not where we typically are looking for supernova. Most of our SNR searches are focused on the plane of the Galaxy toward the Milky Way’s core where we’d expect to find the densest concentration of older and exploded stars. But Hoinga was found at high latitudes off the plane of the Galaxy.
Furthermore, Hoinga hides in the sky because it’s so large. At this scale, the SNR is difficult to distinguish from other large structures of dust and gas that make up the Galaxy known as the “Galactic Cirrus.” It’s like trying to see an individual cloud in an overcast sky. The Galactic Cirrus also outshines Hoinga in radio light, often used to search for SNRs, forcing Hoinga to hide in the background. Cross referencing with older radio sky surveys, the research team determined Hoinga had been observed before but was never identified as an SNR due to its comparatively faint glow in radio. Here eROSITA has an advantage as it sees X-rays. Hoinga shines brighter in X-ray light than the Galactic Cirrus allowing it to stand out from the Galaxy to be discovered.
Tyson’s latest book “Cosmic Queries” covers the gamut from early Earth’s pond scum to potential multiverses to out-of-the-box ideas about the potential that we live in a false vacuum cosmos.
After the past year’s pandemic pall, it’s nice to be reminded that we remain inextricably connected to the cosmos beyond Earth’s atmosphere. In the new book “Cosmic Queries: StarTalk’s Guide to Who We Are, How We Got Here, and Where We’re Going,” astrophysicist and StarTalk podcast host Neil DeGrasse Tyson, along with George Mason University physics professor James Trefil, clearly remind us of our cosmic legacy.
Tyson, Director of New York City’s Hayden Planetarium at the American Museum of Natural History, is well known for his ability to provoke the public into thinking harder about our place in the cosmos. And “Cosmic Queries” does just that. Tyson and Trefil succinctly lead the reader through almost every aspect of cosmic history while addressing age-old questions with new verve.
Designed with a wealth of graphic and color images with pithy captions, the book is also peppered with amusing tweets from Tyson’s own Twitter account over the last decade. The book and his tweets touch on some of StarTalk’s recurring themes, such as Why is the universe the way it is? Are we alone? And how it all began and how it might all end?
In my last post, I talked about the idea of warp drive and whether it might one day be possible. Today I’ll talk about another faster-than-light trick: wormholes.
Wormholes are an old idea in general relativity. It’s based on work by Albert Einstein and Nathan Rosen, who tried to figure out how elementary particles might behave in curved spacetime. Their idea treated particle-antiparticle pairs as two ends of a spacetime tube.
This Einstein-Rosen Bridge would look like a black hole on one end, and an anti-black hole, or white hole, on the other end.