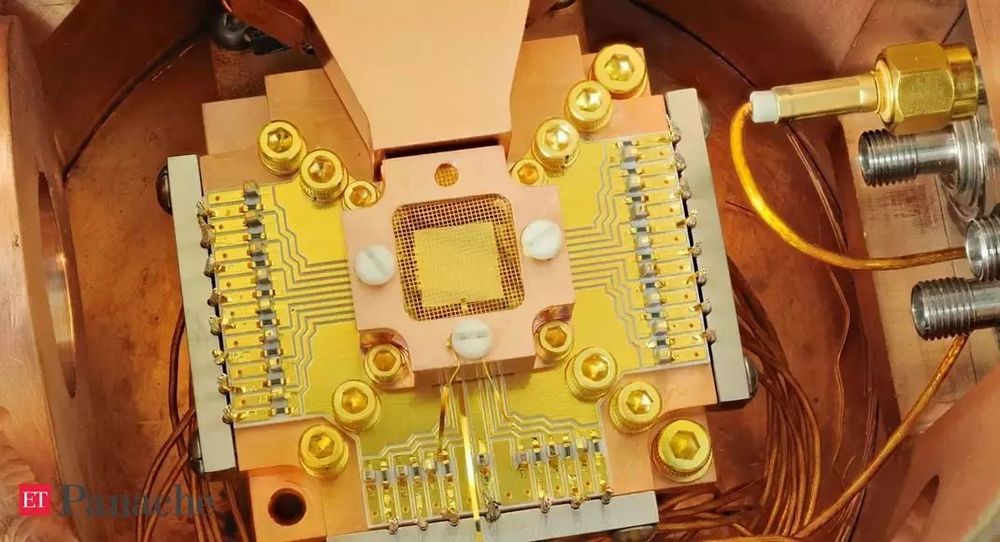
Modern circuitry operates in binaries – switches can either be 0 or 1 – which in turn restricts their computing power to discrete values. Qubits, on the other hand, can hold both values depending on their state, and derives this property from quantum physics. Qubits are modelled on subatomic particles like electrons, giving them an edge over Boolean systems. Quantum computers are difficult to operate, in part due its bulk, power consumption, hardware complexity, and reliance on low temperatures.
Intel’s “hot” qubit technology ought to address the latter concern. These qubits are capable of operating at temperatures higher than 1 Kelvin (−458F / −273K), which is the warmest temperature that quantum computers till now were able to tolerate. Computers in outer space operate at 3 Kelvin. The practical benefits of this breakthrough will manifest itself if Intel can combine quantum hardware and control circuitry on the same chip. It has hitherto been difficult for researchers to separate control electronics for qubits from the qubits themselves owing to the frigid temperature that the latter require to function.
Intel will be hoping that this development will help it fabricate more efficient chips that meld the two parts on the same chip without compromising on fidelity. The commercialization of quantum computing still remains a pipe dream, but large corporations like Google and Intel are paving the way for improvements that could make quantum computers more viable. Even so, make sure you’re wearing a scarf before you go to collect your first quantum computer.