The cause of Alzheimer’s is still not fully understood, but we might be able to vaccinate against it anyway.
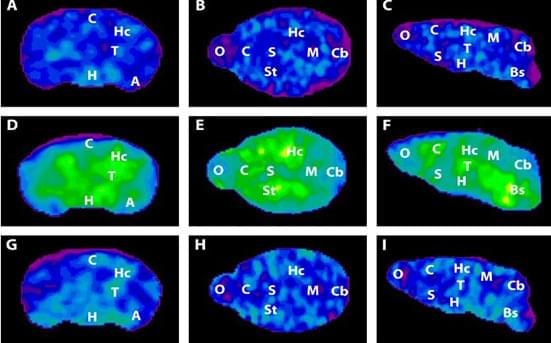
Americans endlessly chatter about what to eat. Low fat, high protein, vegan, ketogenic — but what about electronic? Since the turn of the 21st century, a dedicated group of scientists, engineers, and technologists has been trying to create edible electronics, not necessarily for human nutrition, but rather for medical purposes.
Electronic devices composed of digestible materials that gradually break down in the body over a matter of days could precisely deliver medication inside the body and measure drug uptake. They could monitor symptoms of gastrointestinal disorders and the gut microbiome. They could allow doctors to remotely observe patients’ internal health without a visit to the hospital, further enhancing the telehealth revolution and allowing more people access to healthcare.
As in physics, paradoxes in biology really are just unsolved puzzles. Enter Peto’s paradox. Biologist Richard Peto noticed in the 1970s that mice had a much higher rate of cancer than humans do, which doesn’t make any sense. Humans have over 1,000 times as many cells as mice, and cancer is simply a rogue cell that goes on multiplying out of control. One would expect humans to be more likely to get cancer than smaller creatures such as mice. This paradox occurs across all species, too: blue whales are much less likely to get cancer than humans, even though they have many more cells in their bodies.
Fermi paradox
Named after physicist superstar Enrico Fermi, the Fermi paradox is the contradiction between how likely alien life is in the universe and its apparent absence. Considering the billions of stars in the galaxy like the sun, the many Earth-like planets that must be orbiting some of those stars, the likelihood that some of those planets developed life, the likelihood that some of that life is as intelligent or more intelligent than humanity, the galaxy should be teeming with alien civilizations. This absence led Fermi to pose the question, “Where is everybody?” Some answers to that question are unfortunately a little disturbing.
Erik Verlinde has been compared to Einstein for completely rethinking the nature of gravity.