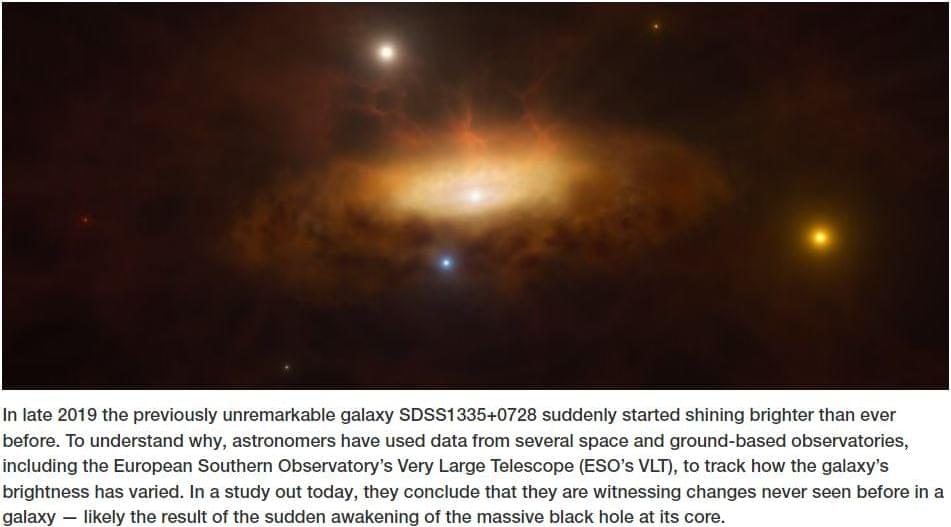
In late 2019 the previously unremarkable galaxy SDSS1335+0728 suddenly started shining brighter than ever before. To understand why, astronomers have used data from several space and ground-based observatories, including the European Southern Observatory’s Very Large Telescope (ESO’s VLT), to track how the galaxy’s @brightness has varied. In a study out today, they conclude that they are witnessing changes never seen before in a galaxy – likely the result of the sudden awakening of the massive black hole at its core.
“Imagine you’ve been observing a distant galaxy for years, and it always seemed calm and inactive,” says Paula Sánchez Sáez, an astronomer at ESO in Germany and lead author of the study accepted for publication in Astronomy & Astrophysics. “Suddenly, its [core] starts showing dramatic changes in brightness, unlike any typical events we’ve seen before.” This is what happened to SDSS1335+0728, which is now classified as having an ‘active galactic nucleus’ (AGN) — a bright compact region powered by a massive black hole — after it brightened dramatically in December 2019 [1].
Some phenomena, like supernova explosions or tidal disruption events — when a star gets too close to a black hole and is torn apart — can make galaxies suddenly light up. But these brightness variations typically last only a few dozen or, at most, a few hundreds of days. SDSS1335+0728 is still growing brighter today, more than four years after it was first seen to ‘switch on’. Moreover, the variations detected in the galaxy, which is located 300 million light-years away in the constellation Virgo, are unlike any seen before, pointing astronomers towards a different explanation.