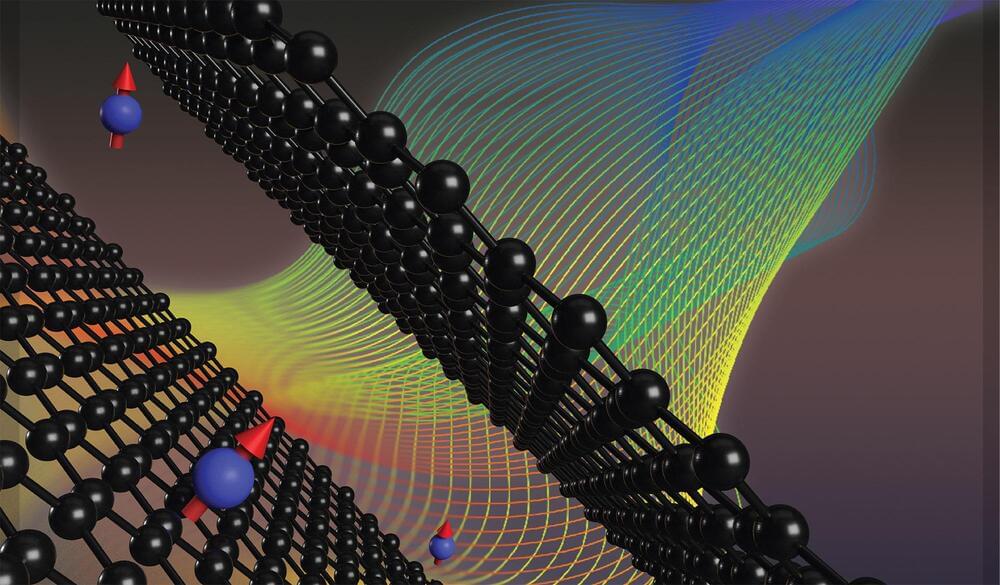
For two decades, physicists have tried to directly manipulate the spin of electrons in 2D materials like graphene. Doing so could spark key advances in the burgeoning world of 2D electronics, a field where super-fast, small and flexible electronic devices carry out computations based on quantum mechanics.
Standing in the way is that the typical way in which scientists measure the spin of electrons—an essential behavior that gives everything in the physical universe its structure—usually doesn’t work in 2D materials. This makes it incredibly difficult to fully understand the materials and propel forward technological advances based on them. But a team of scientists led by Brown University researchers believe they now have a way around this longstanding challenge. They describe their solution in a new study published in Nature Physics.
In the study, the team—which also include scientists from the Center for Integrated Nanotechnologies at Sandia National Laboratories, and the University of Innsbruck—describe what they believe to be the first measurement showing direct interaction between electrons spinning in a 2D material and photons coming from microwave radiation.