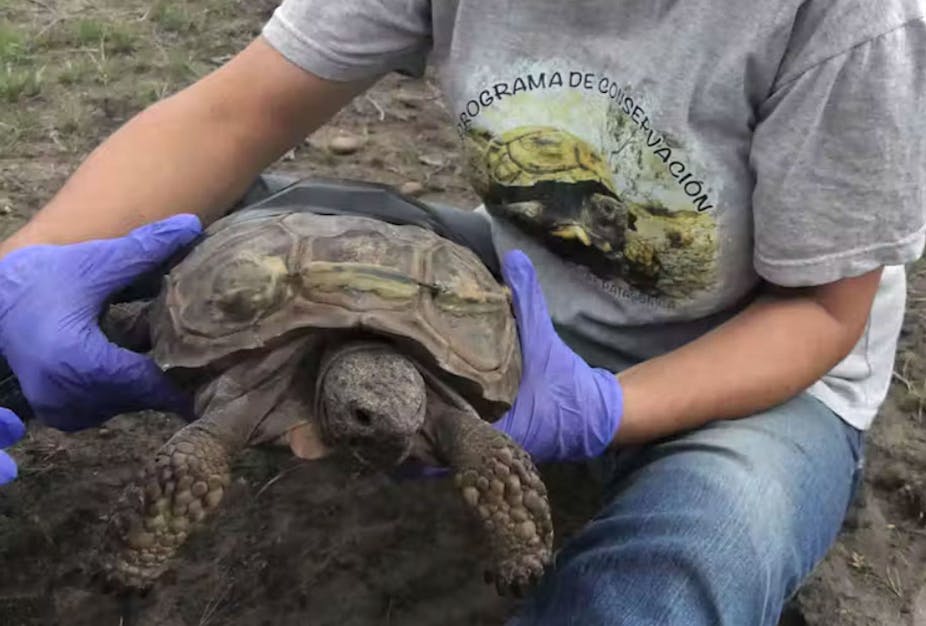
The landscape of artificial intelligence (AI) applications has traditionally been dominated by the use of resource-intensive servers centralized in industrialized nations. However, recent years have witnessed the emergence of small, energy-efficient devices for AI applications, a concept known as tiny machine learning (TinyML).
We’re most familiar with consumer-facing applications such as Siri, Alexa, and Google Assistant, but the limited cost and small size of such devices allow them to be deployed in the field. For example, the technology has been used to detect mosquito wingbeats and so help prevent the spread of malaria. It’s also been part of the development of low-power animal collars to support conservation efforts.
Small size, big impact Distinguished by their small size and low cost, TinyML devices operate within constraints reminiscent of the dawn of the personal-computer era—memory is measured in kilobytes and hardware can be had for as little as US$1. This is possible because TinyML doesn’t require a laptop computer or even a mobile phone. Instead, it can instead run on simple microcontrollers that power standard electronic components worldwide. In fact, given that there are already 250 billion microcontrollers deployed globally, devices that support TinyML are already available at scale.