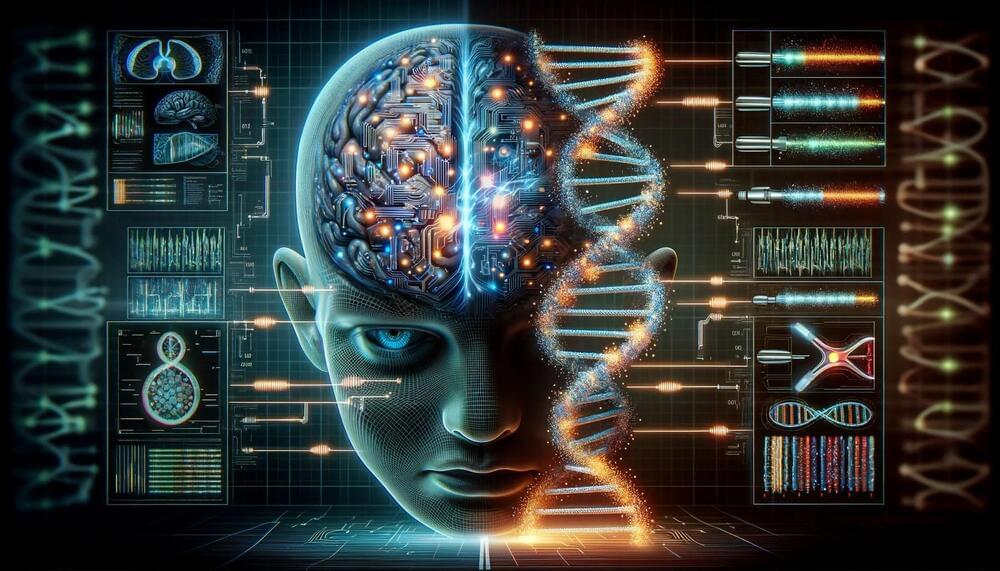
At Oak Ridge National Laboratory (ORNL), quantum biology, artificial intelligence, and bioengineering have collided to redefine the landscape of CRISPR Cas9 genome editing tools. This multidisciplinary approach, detailed in the journal Nucleic Acids Research, promises to elevate the precision and efficiency of genetic modifications in organisms, particularly microbes, paving the way for enhanced production of renewable fuels and chemicals.
CRISPR is adept at modifying genetic code to enhance an organism’s performance or correct mutations. CRISPR Cas9 requires a guide RNA (gRNA) to direct the enzyme to its target site to perform these modifications. However, existing computational models for predicting effective guide RNAs in CRISPR tools have shown limited efficiency when applied to microbes. ORNL’s Synthetic Biology group, led by Carrie Eckert, observed these disparities and set out to bridge the gap.
“A lot of the CRISPR tools have been developed for mammalian cells, fruit flies, or other model species. Few have been geared towards microbes where the chromosomal structures and sizes are very different,” explained Eckert.