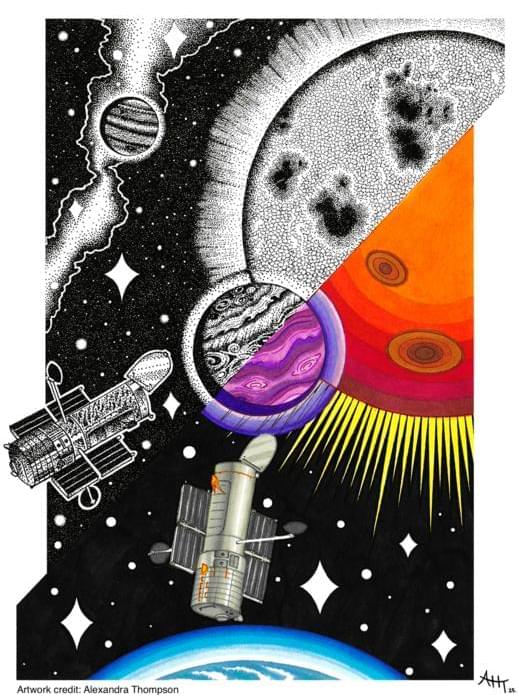
How does a star’s activity influence exoplanet data obtained by scientists? This is what a recent study published in The Astrophysical Journal Supplement Series hopes to address as a team of researchers at University College London (UCL) investigated how stellar activity, specifically star spots, could be “contaminating” exoplanet data, specifically exoplanet atmospheric data. This study has the potential to help astronomers develop more efficient methods for studying exoplanets and their atmospheres, specifically with the number of confirmed exoplanets increasing regularly.
For the study, the researchers used NASA’s Hubble Space Telescope to analyze data from 20 gas giant exoplanets ranging in size between Neptune-like and hot-Jupiter that transited their respective parent stars. To obtain a more complete dataset, the team observed the exoplanets from optical to near-infrared wavelengths. In the end, they discovered a broad range of “stellar contamination”, meaning stellar activity was influencing the exoplanet data, specifically regarding the atmospheric compositions and temperatures. For example, the results indicated that the number of specific molecules had errors as high as 6 orders of magnitude while temperatures had errors as high as 145 percent.
“Hotter, brighter regions (faculae) emit more light and so, for instance, if a planet passes in front of the hottest part of the star, this might lead researchers to over-estimate how large the planet is, as it will seem to block out more of the star’s light, or they might infer the planet is hotter than it is or has a denser atmosphere. The reverse is true if the planet passes in front of a cold starspot, making the planet appear ‘smaller’,” said Alexandra (Alex) Thompson, who is a PhD student in UCL’s Department of Physics & Astronomy and a co-author on the study.