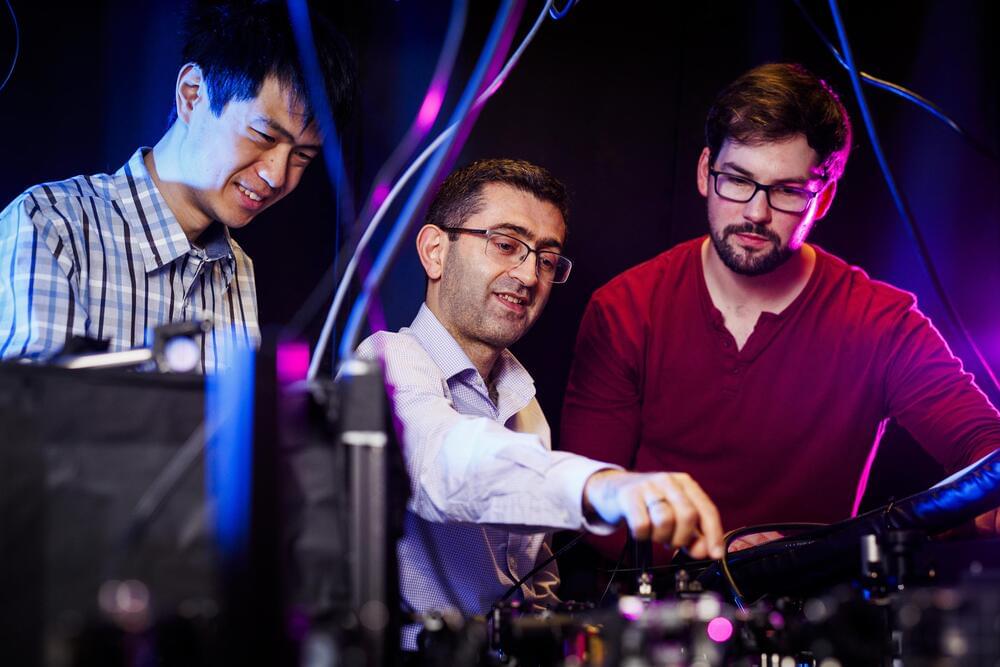
Physicists have created a new and long-lasting magnetic state in a material, using only light. They used a terahertz laser to stimulate atoms in antiferromagnetic materials, which could advance information processing and memory chip technology.
Lighting Up Hidden Magnetism with Terahertz Pulses: A New Frontier in Quantum Materials.
Imagine being able to control the magnetic properties of materials with flashes of light, unlocking states that last long after the light disappears. This groundbreaking approach to quantum materials is at the forefront of condensed-matter physics, offering tantalizing possibilities for future technologies.
In a recent study, researchers discovered a way to create a long-lived magnetic state in the layered material FePS₃ using terahertz light pulses. Typically, materials return to their original state almost immediately after light-induced changes. However, in this case, the induced magnetization persists for over 2.5 milliseconds—an eternity in the quantum world.
The key lies in the material’s proximity to a critical point—its antiferromagnetic transition temperature, where the usual magnetic order starts to fluctuate dramatically. These fluctuations, akin to a system in delicate balance, seem to amplify the material’s response to light, stabilizing the new magnetic state.
By combining advanced computational methods with experiments, the researchers identified that terahertz light excites specific atomic vibrations, subtly shifting interactions between magnetic atoms. Near the critical temperature, these shifts create conditions favoring a stable, magnetized state.
This discovery isn’t just about extending magnetism’s lifespan; it opens the door to manipulating quantum materials in entirely new ways. Regions near critical points, where order teeters on the edge of chaos, could harbor hidden “metastable” states—potentially leading to breakthroughs in memory devices, sensors, and beyond.