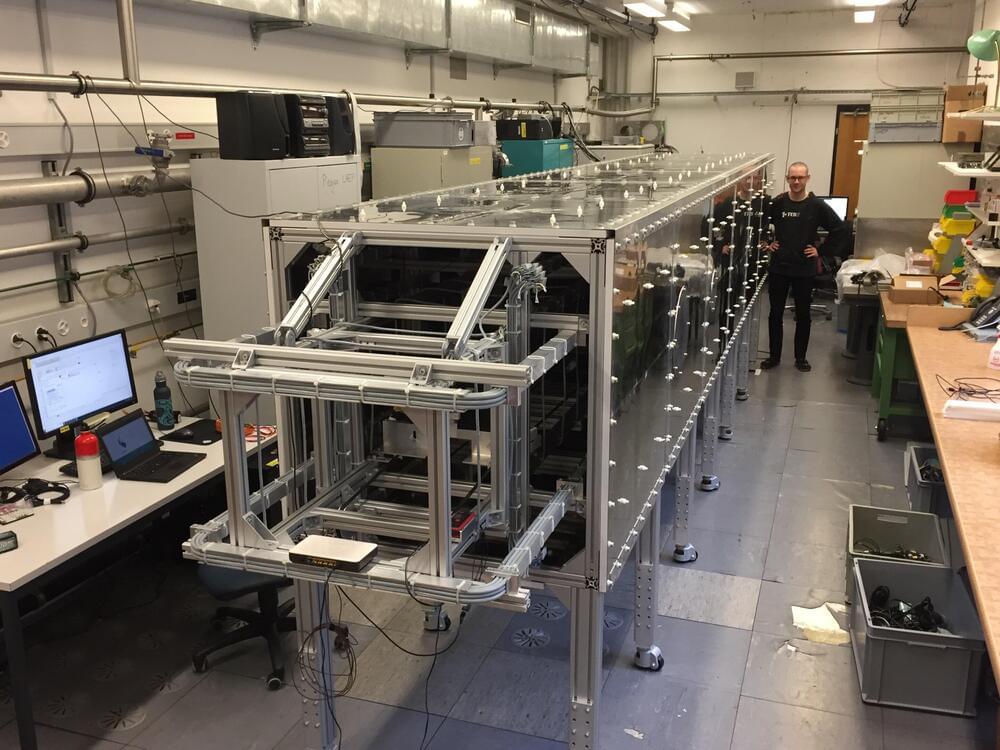
Cosmological observations of the orbits of stars and galaxies enable clear conclusions to be drawn about the attractive gravitational forces that act between the celestial bodies.
The astonishing finding: Visible matter is far from sufficient for being able to explain the development or movements of galaxies. This suggests that there exists another, so far unknown, type of matter. Accordingly, in the year 1933, the Swiss physicist and astronomer Fritz Zwicky inferred the existence of what is known now as dark matter. Dark matter is a postulated form of matter which isn’t directly visible but interacts via gravity, and consists of approximately five times more mass than the matter with which we are familiar.
Recently, following a precision experiment developed at the Albert Einstein Center for Fundamental Physics (AEC) at the University of Bern, an international research team succeeded in significantly narrowing the scope for the existence of dark matter. With more than 100 members, the AEC is one of the leading international research organizations in the field of particle physics. The findings of the team, led by Bern, have now been published in Physical Review Letters.