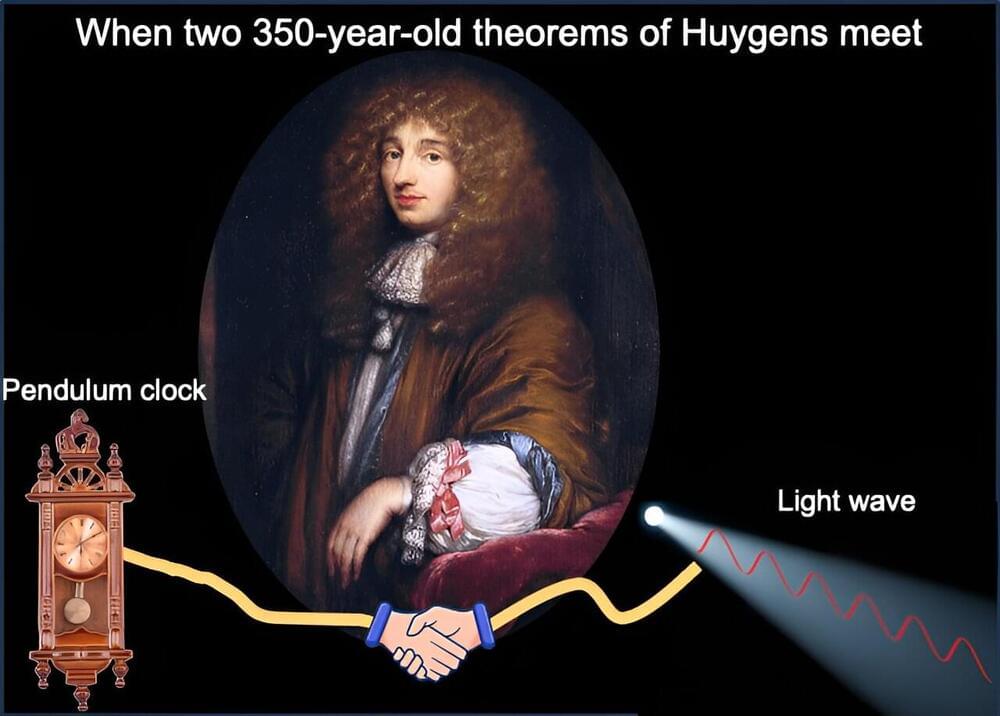
Since the 17th century, when Isaac Newton and Christiaan Huygens first debated the nature of light, scientists have been puzzling over whether light is best viewed as a wave or a particle—or perhaps, at the quantum level, even both at once. Now, researchers at Stevens Institute of Technology have revealed a new connection between the two perspectives, using a 350-year-old mechanical theorem—ordinarily used to describe the movement of large, physical objects like pendulums and planets—to explain some of the most complex behaviors of light waves.
The work, led by Xiaofeng Qian, assistant professor of physics at Stevens and reported in the August 17 online issue of Physical Review Research, also proves for the first time that a light wave’s degree of non-quantum entanglement exists in a direct and complementary relationship with its degree of polarization. As one rises, the other falls, enabling the level of entanglement to be inferred directly from the level of polarization, and vice versa. This means that hard-to-measure optical properties such as amplitudes, phases and correlations—perhaps even these of quantum wave systems—can be deduced from something a lot easier to measure: light intensity.
“We’ve known for over a century that light sometimes behaves like a wave, and sometimes like a particle, but reconciling those two frameworks has proven extremely difficult,” said Qian “Our work doesn’t solve that problem—but it does show that there are profound connections between wave and particle concepts not just at the quantum level, but at the level of classical light-waves and point-mass systems.”