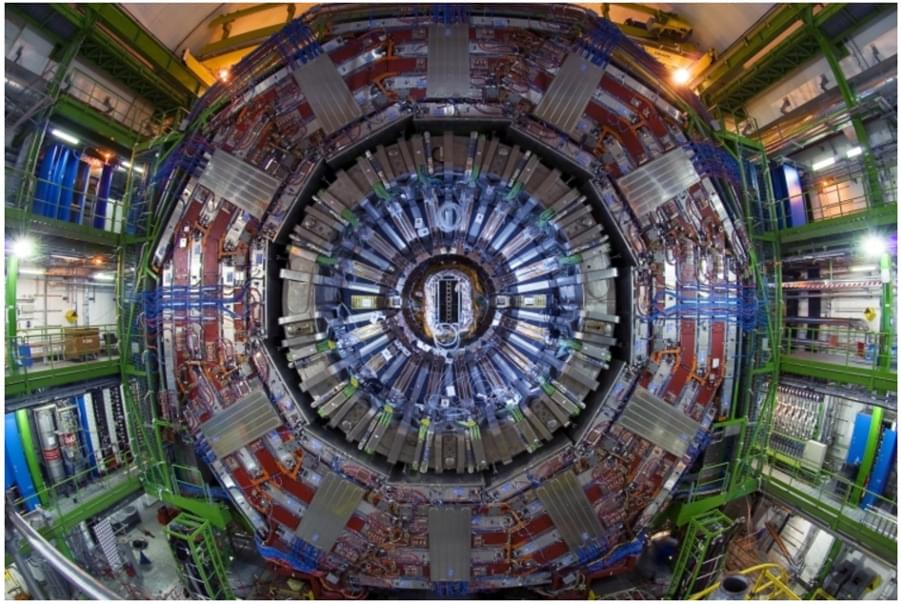
The universe is governed by four known fundamental forces: gravity, electromagnetism, the weak force, and the strong force. The strong force is responsible for dynamics on an extremely small scale, within and between the individual nucleons of atomic nuclei and between the constituents – quarks and gluons – that make up those nucleons. The strong force is described by a theory called Quantum Chromodynamics (QCD). One of the key details of this theory, known as “asymptotic freedom”, is responsible for both the subatomic scale of the strong force and the significant theoretical difficulties that the strong force has presented to physicists over the past 50 years.
Given the complexity of the strong force, experimental physicists have often led the research frontier and made discoveries that theorists are still trying to describe. This pattern is distinct from many other areas of physics, where experimentalists mostly search for and confirm, or exclude, theoretical predictions. One of the QCD areas where experimentalists have led progress is in the description of the collective behavior of systems with many bodies interacting via the strong force. An example of such a system is the quark-gluon plasma (QGP). A few microseconds after the Big Bang, the universe is supposed to have existed in such a state. The way the universe evolved in these brief moments and the structure that subsequently developed over billions of years is studied, in part, through experimental research on collective QCD effects. This briefing describes a recent exciting development in that research. To better understand the results, we begin with a series of analogies.
Imagine you are on a large university campus. You observe student movements in the middle of a busy exam period and find that the number of students entering the library in the morning is related to the number of students leaving in the evening. Perhaps this indicates some conserved quantity, like the number of students at the school. Each student in the library wants enough room to lay out their supplies and textbooks and get comfortable while studying. The library is nearly full and the students are evenly distributed across all the floors and halls of the library to ensure they have ample space. Recognizing and quantifying correlations like these can be useful for studying collective systems. By counting students “here” you can predict how many students are “there”, or by counting students “now” you can predict how many students you will get “later”. In this example, you may have insight into basic temporal and spatial correlations.