For the first time, physicists have calculated exactly what kind of singularity lies at the center of a realistic black hole.
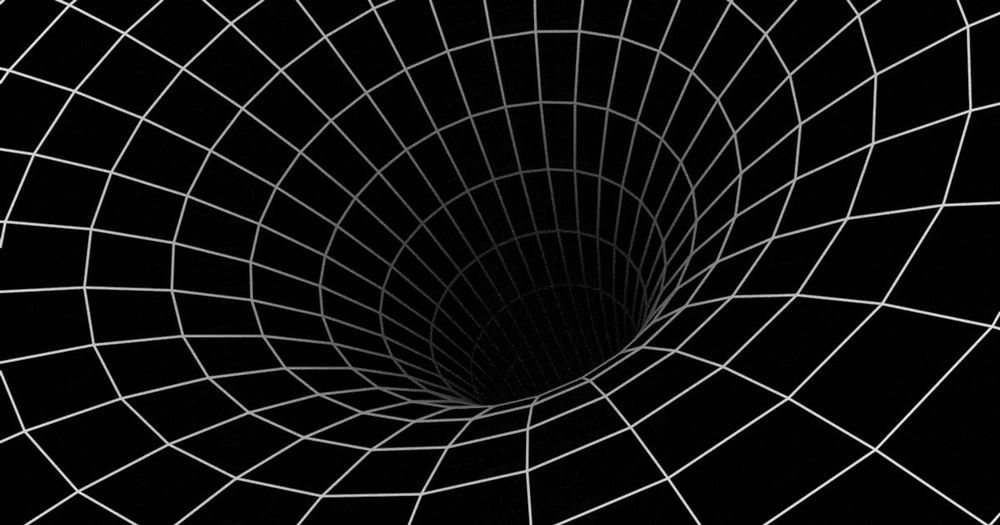
The universe is filled with billions of galaxies and trillions of stars, along with nearly uncountable numbers of planets, moons, asteroids, comets and clouds of dust and gas – all swirling in the vastness of space.
But if we zoom in, what are the building blocks of these celestial bodies, and where did they come from?
Hydrogen is the most common element found in the universe, followed by helium; together, they make up nearly all ordinary matter. But this accounts for only a tiny slice of the universe — about 5%. All the rest is made of stuff that can’t be seen and can only be detected indirectly. [From Big Bang to Present: Snapshots of Our Universe Through Time].
What – one vast, ancient and mysterious universe isn’t enough for you? Well, as it happens, there are others. Among physicists, it’s not controversial. Our universe is but one in an unimaginably massive ocean of universes called the multiverse.
If that concept isn’t enough to get your head around, physics describes different kinds of multiverse. The easiest one to comprehend is called the cosmological multiverse. The idea here is that the universe expanded at a mind-boggling speed in the fraction of a second after the big bang. During this period of inflation, there were quantum fluctuations which caused separate bubble universes to pop into existence and themselves start inflating and blowing bubbles. Russian physicist Andrei Linde came up with this concept, which suggests an infinity of universes no longer in any causal connection with one another – so free to develop in different ways.
Cosmic space is big – perhaps infinitely so. Travel far enough and some theories suggest you’d meet your cosmic twin – a copy of you living in a copy of our world, but in a different part of the multiverse. String theory, which is a notoriously theoretical explanation of reality, predicts a frankly meaninglessly large number of universes, maybe 10 to the 500 or more, all with slightly different physical parameters.
Supermassive black holes exist at the center of most galaxies, and our Milky Way is no exception. But many other galaxies have highly active black holes, meaning a lot of material is falling into them, emitting high-energy radiation in this “feeding” process. The Milky Way’s central black hole, on the other hand, is relatively quiet. New observations from NASA’s Stratospheric Observatory for Infrared Astronomy, SOFIA, are helping scientists understand the differences between active and quiet black holes.
Before his death, Stephen Hawking submitted a research paper that predicts the end of the world.
Just two weeks before his death, renowned physicist Stephen Hawking submitted a research paper that suggests parallel universes and predicts the end of this one.
Hawking and his co-author Thomas Hertog published their research in “A Smooth Exit from Eternal Inflation,” detailing how scientists may also be able to detect other universes using a spaceship. According to Hertog, Hawking finished writing the paper from his deathbed, leaving behind a final legacy that is worthy of the Nobel Prize.
“He has often been nominated for the Nobel and should have won it. Now he never can,” he told the Sunday Times.
Before now, scientists did not think it was possible for a stellar black hole to have a mass larger than 20 times that of the sun, an approximation based on their understanding of the way stars evolve and die in the Milky Way.
But that assumption was metaphorically crushed in the gravity of a “monster” black hole that a group of Chinese-led international scientists discovered inside our own galaxy. The hole has a mass 70 times that of the sun, researchers said in their study published in the journal Nature.
The problem of surviving the end of the observable universe may seem very remote, but there are several reasons it may be important now: a) we may need to define soon the final goals of runaway space colonization and of superintelligent AI, b) the possibility of the solution will prove the plausibility of indefinite life extension, and с) the understanding of risks of the universe’s end will help us to escape dangers like artificial false vacuum decay. A possible solution depends on the type of the universe’s ending that may be expected: very slow heat death or some abrupt end, like a Big Rip or Big Crunch. We have reviewed the literature and identified several possible ways of survival the end of the universe, and also suggest several new ones. There are seven main approaches to escape the end of the universe: use the energy of the catastrophic process for computations, move to a parallel world, prevent the end, survive the end, manipulate time, avoid the problem entirely or find some meta-level solution.